Understanding the Complexity of Biological Systems
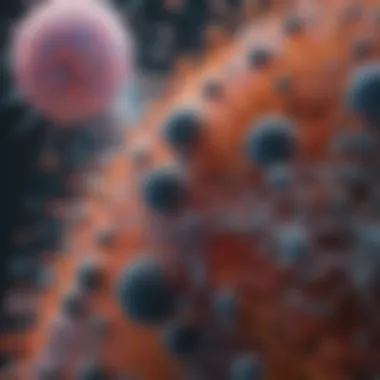
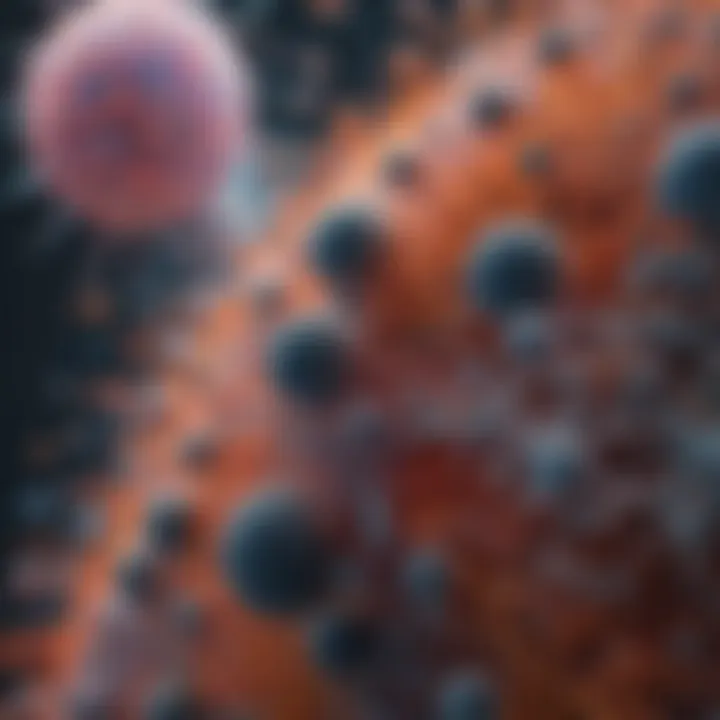
Intro
Biological systems are, in a word, marvelously intricate. They encompass a vast range of organized elements that work together to sustain life, from the tiniest cell to entire ecosystems. Examining these systems isn't just an academic exercise; it's a vital journey that leads us to a deeper understanding of life itself. Whether you’re delving into the depths of cellular processes or observing the grand tapestry of an ecosystem at work, each layer of organization reveals a unique interplay that is essential for survival. This article endeavours to unravel these complexities, shining light on the key principles and interrelationships that define biological systems.
The concept of biological systems can seem overwhelming at first, but when broken down into its core components, patterns and connections emerge. Through various levels of biological organization, we can better appreciate how life thrives and adapts. This understanding isn't merely of scholarly interest; it has practical implications in fields such as medicine, environmental science, and conservation efforts.
"Understanding biological systems equips us with the knowledge to address pressing global issues, like climate change and habitat destruction."
Exploration of biological systems stretches beyond the laboratory or classroom; it opens pathways to real-world applications. By the end of our discussion, we aim to illuminate how these interconnected systems function, adapt, and sometimes struggle against the influence of human activities.
In the sections that follow, we will delve into the structure and functionality of biological systems, shedding light on their individual and collective roles. Let's embark on this enlightening exploration together.
Understanding Biological Systems
Understanding biological systems is essential for grasping how life functions at all levels, from the tiniest molecules to complex ecosystems. Biological systems are not just collections of parts; rather, they are intricate networks where each component plays a specific role in a grander scheme. Recognizing this complexity allows us to appreciate the delicate balance that sustains life.
When we break down biological systems, we're looking at their organization, operation, and interactions. Each level of organization—from molecular to ecosystem—offers unique insights into how life forms maintain themselves and adapt to their environments. The benefits of understanding these systems are manifold:
- It fosters a clearer comprehension of life's processes and the mechanisms behind them.
- It aids in the study of diseases and the development of treatments by elucidating how systems can become unbalanced.
- It triggers curiosity about the interconnectedness of different organisms, prompting questions about biodiversity and ecological health.
For those entering fields like biology, medicine, or ecology, a thorough understanding of biological systems serves as a bedrock for further study. It lays the groundwork for future exploration into specialized areas such as genetics, environmental science, or microbiology.
This knowledge shapes not only academic pursuits but also practical applications in agriculture, conservation, and health industries.
As we dive deeper into these topics, one must consider the numerous factors that influence biological systems, including both intrinsic mechanisms—like genetic makeup and physiological processes—and extrinsic factors, such as environmental changes and human activities. Understanding these variables is crucial for anyone working within these fields.
Levels of Biological Organization
The study of biological systems requires an understanding of how various levels of organization contribute to the complexity and functionality of life. Each rung on this hierarchical ladder plays a vital role in sustaining life, from the microscopic details of molecular interactions to the grand designs of ecosystems. This framework highlights the importance of recognizing that biological phenomena do not exist in isolation; they are intricately interwoven and dependent on each other.
A comprehensive grasp of these levels can aid researchers and students alike in explaining biological processes and appreciating their interconnectedness. It can unveil how changes at one level can ripple through the other levels, affecting the entire system.
Molecular Level
Biomolecules
Biomolecules, the building blocks of life, are crucial components of biological systems. They include proteins, nucleic acids, carbohydrates, and lipids. One standout feature of biomolecules is their diverse structures and functions, which are critical to life's processes. For instance, proteins can serve as enzymes, transport molecules, or play roles in immune responses.
The adaptability of biomolecules makes them a fascinating subject. Their ability to undergo modification and interact with each other allows for a dynamic and flexible system that can respond to environmental changes. This adaptability is particularly beneficial when understanding metabolic pathways and cellular activities, as changes in biomolecular interactions can lead to vastly different outcomes in biological functions.
Proteins
Proteins, a class of biomolecules, are central to nearly every function in a living organism. From catalyzing biochemical reactions to providing structural support, proteins have a key characteristic— specificity. This means they can perform very particular tasks depending on their structure. For this narrative, focusing on enzymes as a unique feature of proteins illustrates their role in catalyzing reactions essential for metabolism.
While proteins are incredibly useful tools in biological mechanisms, they also come with a drawback: they can be sensitive to environmental conditions such as temperature and pH. This sensitivity can lead to denaturation, whereby proteins lose their functional shape and, thus, their ability to perform tasks effectively, presenting challenges in biological processes.
Nucleic Acids
Nucleic acids, primarily DNA and RNA, are fundamental for the storage and expression of genetic information. Their specific structure— the double helix for DNA—allows for stable storage of genetic material, while the single-stranded RNA provides versatility in its role as a messenger and a catalyst in biological reactions.
This unique feature of nucleic acids makes them indispensable in understanding hereditary information transfer and cellular function regulation. Nevertheless, mutations and errors in nucleic acid sequencing can lead to significant disadvantages, such as genetic disorders or malfunctioning cellular processes, emphasizing their critical role in maintaining life's integrity.
Cellular Level
Cell Structure
The structure of cells is the fundamental unit of life. Understanding the cellular level provides insights into how organismal life is organized and functions. Key characteristics of cell structure, such as the cell membrane, cytoplasm, and organelles, contribute to the cell's overall functionality.
One unique feature of cell structure is the compartmentalization of organelles, which allows for specialized functions to occur simultaneously within a single cell. This compartmentalization benefits the cell by increasing efficiency in carrying out various biochemical processes. However, the disadvantage lies in the potential for issues to arise if cell structure is compromised, potentially leading to cell death.
Cell Division
Cell division is a fundamental process for growth and reproduction in organisms. Through mechanisms such as mitosis and meiosis, cells can proliferate and pass on genetic information to the next generation. The key characteristic of cell division is its ability to ensure that genetic material is accurately distributed to daughter cells, which is vital for maintaining genetic continuity.
While cell division is essential, it can also have disadvantages, particularly when errors occur in the process. These mistakes can lead to cancer or genetic abnormalities, emphasizing the importance of precise regulation of this process.
Cell Signaling
Cell signaling is an intricate system of communication that directs cellular activities. Through signaling molecules and pathways, cells can respond to changes in their environment, ensuring that the organism functions cohesively. The key characteristic of cell signaling lies in its specificity, allowing cells to communicate effectively and respond appropriately to stimuli.
However, the complexity of cell signaling can lead to issues such as miscommunication or overactive signaling pathways, which can result in disease states. These disadvantages highlight the delicate balance required in cellular communication for maintaining health.
Tissue Level
Types of Tissues
Different types of tissues play crucial roles within an organism and are classified into four primary categories: epithelial, connective, muscle, and nervous. Each type has its own key characteristic—for instance, epithelial tissue serves as a protective barrier, while connective tissue provides support and structure.
A unique feature of these tissues is their specialized functions tailored for specific needs, greatly benefiting the organism. The disadvantage, however, is that damage to one type of tissue can affect the overall function of the system, demonstrating the importance of interdependence among tissue types.
Tissue Function
The function of tissues reflects their intricacies and how they contribute to the greater organism. For example, muscle tissue is responsible for movement, while nervous tissue facilitates communication. The distinctive function of each tissue type underscores the overall efficiency of biological systems.
Nevertheless, dysfunction in tissue function—whether through disease or injury—can lead to serious health issues. This highlights how the successful operation of each tissue type is essential for the organism's wellness.
Tissue Regeneration
Tissue regeneration showcases the remarkable ability of some tissues to repair themselves after injury. Certain tissues, like epithelial and connective tissues, exhibit higher regenerative capacity. This characteristic is particularly beneficial for survival, as it allows for quick recovery and minimal disruption to bodily functions.
On the flip side, regeneration is not universally applicable across all tissues. For instance, nervous tissues have limited regenerative capabilities, which can lead to long-term deficits if damaged, revealing a critical limitation in biological systems.
Organ Level
Organ Structure
Organ structure pertains to the intricate design of various organs that work together to form systems. Each organ has a specific arrangement of tissues that aids its function; for instance, the heart is composed of muscle and connective tissue, enabling it to pump blood efficiently.
A unique feature of organ structure lies in its design for functionality, enhancing performance in specific tasks. However, this specialization comes with disadvantages as damage to any organ can dramatically impact overall system performance.
Organ Systems
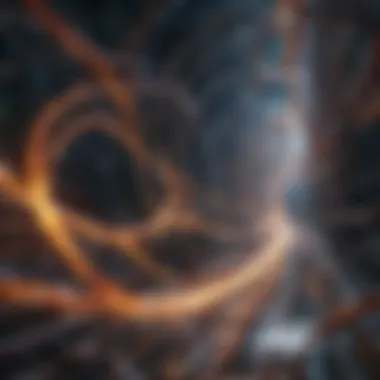
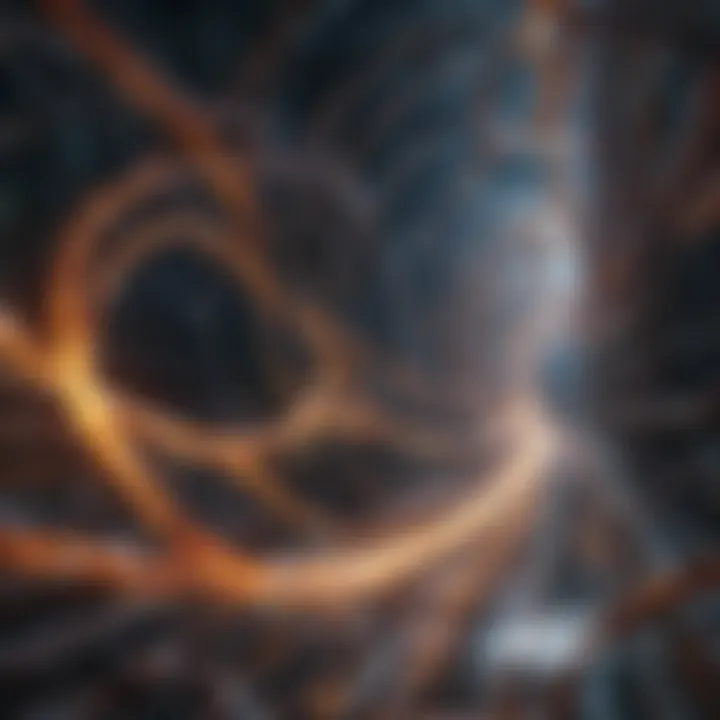
Organ systems comprise multiple organs working harmoniously to perform complex functions, such as the circulatory system facilitating blood flow. The key characteristic here is organization, which ensures that various organs coordinate effectively.
Despite their efficiency, organ systems are susceptible to systemic failures—should one organ falter, it could compromise the entire system, demonstrating the need for all parts to function optimally.
Inter-organ Communication
Inter-organ communication enables different organs to send signals to one another, maintaining homeostasis within the body. The key aspect is that these communications often utilize hormonal signals or neural pathways, highlighting their interdependence.
Yet, this communication system can become disrupted due to disease or environmental challenges, leading to significant health issues. This risk sheds light on the fragility of these complex interactions.
Organism Level
Homeostasis
Homeostasis refers to the internal balance that organisms must maintain to function correctly. The key characteristic of homeostasis lies in its mechanisms to self-regulate bodily functions despite external changes. This capacity allows organisms to thrive in various conditions.
However, one disadvantage is that failure in maintaining homeostasis can result in dysfunction or disease, illuminating the critical nature of this balance.
Growth and Development
Growth and development encompass the processes that allow organisms to mature and adapt. The uniqueness of this progression lies in its orderly stages, from a single cell to a fully formed organism.
A downside exists in cases of abnormal growth patterns or developmental disorders, which can hinder an organism's overall functionality, emphasizing how growth and development must be properly regulated.
Reproductive Systems
The reproductive systems are responsible for perpetuating species. Highlighting their significance, reproductive systems facilitate genetic diversity through mechanisms such as sexual reproduction.
However, one disadvantage of these systems is their complexities—difficulties in reproductive health can lead to challenges in sustaining populations, which underlines their vital role in biological continuity.
Population Level
Population Dynamics
Population dynamics involves the study of changes in populations over time. Recognizing trends in birth, death, immigration, and emigration can provide insights into the factors affecting population stability.
One of the defining features of population dynamics is the ability to predict changes, which is essential for conservation efforts. However, unpredictable factors like climate change can significantly disrupt these patterns, underscoring its challenges.
Species Interactions
Species interactions, including predation, competition, and symbiosis, highlight the interconnectedness of ecosystems. The key characteristic of these interactions is their capacity to shape community structures.
Yet, the flipside to such interactions is potential imbalance—any sudden changes, such as invasive species introduction, can disrupt existing relationships, making this a delicate balance to maintain.
Genetic Variation
Genetic variation contributes to a population's resilience, ensuring adaptability in changing environments. The uniqueness lies in its role in evolution and natural selection.
However, a disadvantage occurs when a population experiences significant reductions in genetic variation, leading to vulnerability to environmental changes, thereby threatening species survival.
Community Level
Community Structure
Community structure refers to the organization of different species living together in a defined area. The key feature is the complexity arising from diverse interactions between species, which can lead to unique ecological outcomes.
However, loss of biodiversity can disrupt this structure, highlighting the importance of conservation efforts to maintain balanced ecosystems.
Ecological Niche
The ecological niche illustrates the role a species plays in its environment, describing its habitat and interactions. Its distinctiveness lies in how it contributes to ecosystem function and stability.
Nevertheless, niche overlap can result in competition, affecting species survival, prompting attention to species conservation and biodiversity maintenance.
Biodiversity
Biodiversity, the variety of life within an ecosystem, is vital for ecosystem resilience. The key characteristic is that it supports ecosystem services that benefit all life forms.
Yet human activities, like urbanization and deforestation, can threaten biodiversity, underscoring the need for mindful stewardship of natural resources.
Ecosystem Level
Energy Flow
Energy flow refers to the transfer of energy through food chains within ecosystems. The key characteristic is its efficiency and the manner in which energy diminishes at higher trophic levels.
However, disruptions—such as overfishing or habitat destruction—can severely impact energy flow, threatening the stability of entire ecosystems.
Nutrient Cycling
Nutrient cycling is the recycling of essential nutrients through various biological and geological processes. This concept is crucial as it sustains ecosystem productivity.
Yet, disturbances in this cycle, often due to pollution and land-use changes, can lead to nutrient depletion, impacting ecosystem health.
Human Impact
Human impact summarizes the various ways human activities can alter biological systems. Acknowledging the key characteristic here emphasizes the scale and speed at which these changes can occur.
Nevertheless, this impact presents challenges in ecological conservation efforts, highlighting the pressing need for sustainable practices to mitigate adverse effects of human actions.
Key Processes in Biological Systems
The processes that drive biological systems are essential to understanding how life thrives and adapts. Metabolism, homeostasis, reproduction, and adaptation and evolution are among the key processes responsible for maintaining the balance of life. Each of these processes intertwines, influencing not only individual organisms but also entire ecosystems. A solid grasp of these elements lays the groundwork for any deeper exploration into the complexities of biological systems.
Metabolism
Metabolism encompasses all biochemical reactions occurring within living organisms, vital for maintaining life. Two significant aspects of metabolism are catabolism and anabolism, which work together to provide energy and build cellular components.
Catabolism
Catabolism refers to the breakdown of complex molecules into smaller ones, releasing energy in the process. This energy can be stored or used immediately for cellular activities. A key characteristic of catabolism is its role in energy release. It's a commonly favored topic in biological discussions due to its clear connection to energy production. A unique feature of catabolism is that it allows organisms to recycle nutrients effectively.
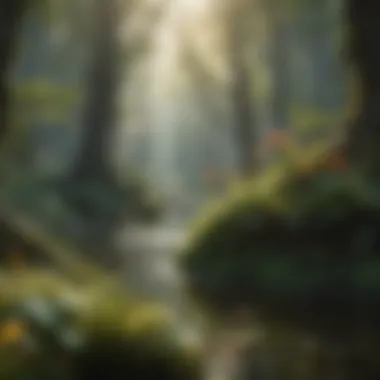
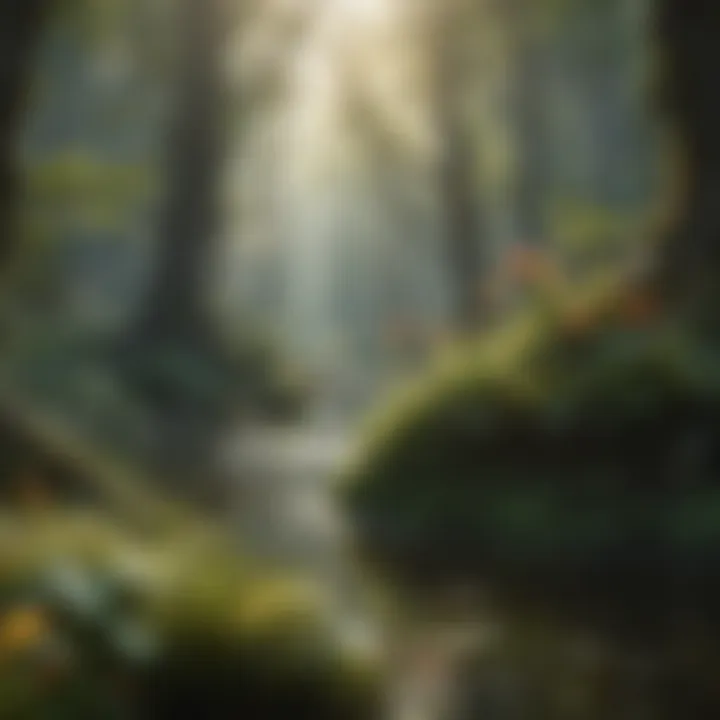
- Advantages: Provides necessary energy for various cellular processes.
- Disadvantages: If mismanaged, it can lead to the depletion of vital resources within cells.
Anabolism
Anabolism, the opposite of catabolism, is about building complex molecules from simpler ones. It requires energy input, which is often derived from the energy released during catabolism. The synthesis of proteins and nucleic acids are examples of anabolic processes, making this a critical area of focus in biological studies. This process is beneficial as it contributes to growth, reproduction, and tissue repair, presenting a solid case for its significance in the field.
- Advantages: Essential for growth and recovery in living organisms.
- Disadvantages: Energy-intensive, requiring substantial resources to sustain.
Enzyme Activity
Enzymes serve as catalysts in metabolic pathways, substantially accelerating chemical reactions vital for life. Their key characteristic is specificity; each enzyme typically works on a particular substrate, ensuring efficiency in metabolic processes. This specificity makes enzyme activity a popular subject in studies related to metabolism. Enzymes have a unique feature: they lower the activation energy required for reactions to occur, driving metabolic processes with remarkable precision.
- Advantages: Speed up reactions and lower energy requirements for metabolic processes.
- Disadvantages: Their activity can be hindered by environmental factors such as pH and temperature, which might limit effectiveness in certain conditions.
Homeostasis
Homeostasis is the process through which organisms regulate their internal environments to maintain stability, despite changes in their external conditions. It's crucial for survival as it ensures optimal conditions for cellular processes and overall functioning.
Feedback Mechanisms
Feedback mechanisms, particularly negative feedback loops, are central to maintaining homeostasis. They involve sensing a change and responding to counteract it. This feature is significant as it allows organisms to correct deviations from a set point, like body temperature. Feedback mechanisms are beneficial as they help sustain life by maintaining equilibrium.
- Advantages: Quick adjustments ensure survival and optimal performance.
- Disadvantages: Over-reliance on these mechanisms can lead to systemic failures if not balanced properly.
Regulatory Pathways
Regulatory pathways describe how cellular signals and responses maintain homeostasis. They are essential for managing diverse processes such as metabolism, growth, and stress responses. The intricate networks of regulatory pathways highlight the complexity of biological systems. Their unique characteristic is adaptability, allowing cells to respond dynamically to varying internal and external stimuli.
- Advantages: Provide comprehensive control over biological processes.
- Disadvantages: Complexity can lead to inefficiencies or errors in biological responses.
Adaptation
Adaptation refers to the changes that enable organisms to survive in their environments, defined by both physiological and behavioral responses. The characteristic of adaptation lies in its evolutionary nature, showcasing how organisms become better suited to their environments over time. This dimension is fundamental as it portrays the interconnectedness of evolution and biology.
- Advantages: Enhances survival chances in changing environments.
- Disadvantages: Adaptation can lead to reduced genetic diversity in certain scenarios.
Reproduction
Reproduction is fundamental for the continuation of species, and it encompasses both asexual and sexual reproduction methods. Understanding these processes provides insights into genetic variation and evolution.
Asexual vs Sexual Reproduction
Asexual reproduction involves a single organism creating a clone of itself, while sexual reproduction requires the contribution of genetic material from two parents. Each method has its unique attributes; asexual reproduction is rapid, allowing for quick population increases, making it a beneficial choice in stable environments.
- Advantages of Asexual: Efficient and minimizes resource use.
- Disadvantages of Asexual: Low genetic diversity can hinder adaptation.
- Advantages of Sexual: High genetic diversity enhances adaptability.
- Disadvantages of Sexual: More resource-intensive and requires more time.
Life Cycles
Life cycles illustrate the various stages through which an organism develops, from initiation to maturity. They are pivotal in understanding reproduction and species continuity. The characteristic of life cycles lies in their complexity and variance across species. A beneficial aspect is providing a framework for studying growth and development throughout life stages.
- Advantages: Enable organisms to adapt at different life stages.
- Disadvantages: Some life stages may be more vulnerable to environmental stressors.
Genetic Diversity
Genetic diversity is the variation in genes within a population, a key contributor to overall health and adaptability. It underpins the resilience of species and their ability to survive environmental stresses. This characteristic of genetic diversity helps illustrate the evolutionary mechanisms at work in biological systems, encouraging focus on its importance within biological studies.
- Advantages: Increases resilience and adaptability to changing conditions.
- Disadvantages: Lower genetic diversity can lead to greater vulnerability.
Adaptation and Evolution
Adaptation and evolution represent the processes through which species change over time through natural selection, speciation, and various evolutionary mechanisms. These processes underscore the dynamic nature of life.
Natural Selection
Natural selection involves the differential survival and reproduction of individuals due to advantageous traits. Its key characteristic is the survival of the fittest, a principle that offers a straightforward lens through which to view evolutionary change. By focusing on natural selection, researchers can better understand how traits persist or disappear in populations.
- Advantages: Promotes adaptation and survival of the species.
- Disadvantages: Can result in the loss of less favorable traits.
Speciation
Speciation describes the process through which new species arise. It typically occurs when populations become isolated and evolve due to different selective pressures. A unique feature here is that speciation helps explain biodiversity, a central theme in ecology and evolutionary biology.
- Advantages: Leads to increased diversity and ecological niches.
- Disadvantages: Can take considerable time and requires specific conditions.
Evolutionary Mechanisms
Various mechanisms drive evolution, including genetic drift, mutation, and gene flow, each contributing to the diversity of life. The key characteristic of these mechanisms lies in their complexity and interplay. Understanding these mechanisms not only illuminates evolutionary processes but also enhances comprehension of how species evolve in response to environmental changes.
- Advantages: Helps explain the adaptability of species.
- Disadvantages: The complexity of interactions can yield unpredictable outcomes.
Human Influence on Biological Systems
Human activities have far-reaching consequences on biological systems across the globe. Our relentless expansion into natural habitats and the intricate relationships that exist within ecosystems have led to significant changes, many of which are detrimental to the very life forms we depend on. By understanding the multifaceted impacts of our presence, we can better gauge how to shape sustainable futures that ensure the preservation of vital biological systems.
Impact of Urbanization
Habitat Loss
When cities expand and infrastructure develops, natural habitats often vanish, paving the way for urban landscapes. Habitat loss is a pressing issue, as it strips ecosystems of the native species that thrive within them. For example, wetlands are drained for new developments, breaking the delicate balance of local flora and fauna. This consequence is not merely an inconvenience; it systems integrity is crucial for local ecosystems.
A key characteristic of habitat loss includes habitat fragmentation, where large natural spaces dissected with roads and buildings prevent movement and mating, ultimately leading to a decline in genetic diversity. It's a major consideration in this discourse as it underscores the need for urban planners to integrate biodiversity strategies into their designs. While cities cannot be stopped, the loss can be mitigated through intentional green spaces that provide refuge to displaced organisms.
Biodiversity Decline
Following closely on the heels of habitat loss is the biodiversity decline that typically accompanies urban development. The rich tapestry of life that once thrived in a region can see drastic reductions when alien species are introduced, or when native species are pushed to the brink of extinction. This decline indisputably contributes to the article's purpose of understanding human impacts; without biodiversity, ecosystems weaken and can no longer deliver essential services like clean air and water filtration.
The unique feature of biodiversity decline is its ripple effect on ecosystem services. For instance, as pollinators dwindle, crop yields suffer. This illustrates a critical concern that must be noted: the loss of even one species can initiate a cascade of negative outcomes.
Pollution
Pollution, another oft-cited consequence of urbanization, involves the introduction of harmful substances into the natural environment. Contaminants from industrial runoff, plastic waste, and unfiltered emissions compromise the health of ecosystems. Such action not only poisons wildlife but also puts human health at risk. The importance of understanding pollution cannot be overstated; these pollutants are often invisible foes, wreaking havoc on water quality and air purity.
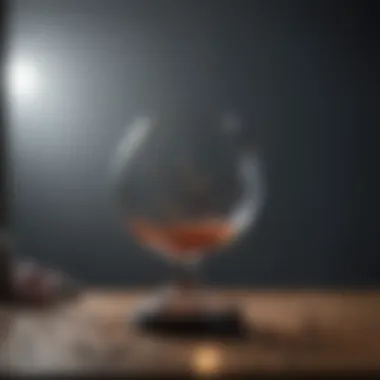
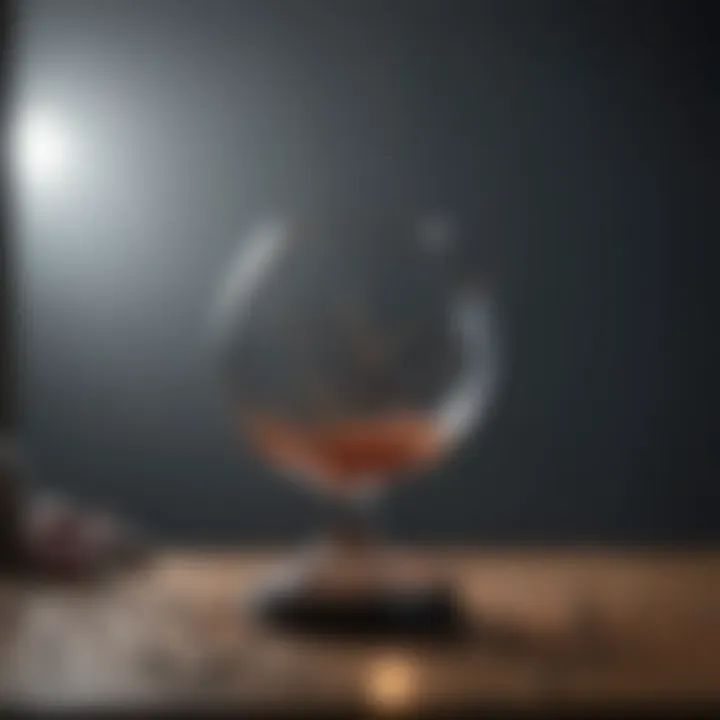
Unique to pollution is its pervasive nature, affecting organisms across all levels from microbes to mammals. While urban areas can promote economic growth, the hidden costs of pollution lead to declining public health, making it a central issue that requires ongoing attention and proactive measures.
Agricultural Practices
Monoculture
The predominance of monoculture in agriculture is a specific practice with vast implications on biological systems. This practice involves cultivating a single crop over a wide area, boasting immediate economic efficiency from reduced labor costs and homogenous harvesting schedules. However, such a choice is perilous for long-term ecological health. This habitat simplification diminishes the variety of life and weakens the soil. Paths of disease can sweep through monoculture fields, affecting entire harvests.
In enhancing the understanding of this article, monoculture stands out as a double-edged sword. It reflects the human desire for control but also illustrates the fragility of ecosystems reliant on diversity.
Pesticides Use
The use of pesticides is another characteristic of modern agriculture that has grave consequences for biological systems. These chemical agents protect crops from pests but often don't discriminate, harming beneficial species and contaminating soil and water supply. The ongoing reliance on pesticides raises concerns regarding their long-term effects—specifically, they lead to a resurgence of pest populations that are resistant to chemical agents. A pertinent point here is how this paves the way for increased chemical usage, creating a cycle of dependency.
Thus, the unique feature of pesticide use lies in its short-term protection coupled with the risk of long-term ecological imbalance.
Soil Degradation
Related to both monoculture and pesticide use is soil degradation, a serious issue that underpins agricultural sustainability. Degrades from erosion, loss of nutrients, and water-logging diminish the earth's ability to sustain plant life, raising alarms about food security. A key characteristic is the erosion of soil quality, not just a loss of nutrients but an entire collapse of structure necessary for healthy plant growth.
Soil degradation speaks volumes about how human practices can undermine the very basis of our food systems. The warning bells are ringing; without intervention, our agricultural land may become increasingly unproductive.
Climate Change Effects
Habitat Alteration
Increasingly, habitat alteration emerges as a result of climate change, reshaping environments faster than species can adapt. Altered rainfall patterns lead to dry spells or flooding, affecting habitats from the smallest streams to vast forests. This change can segregate species, diminishing ecosystem functionality. By drawing in the realms of climate change, this aspect emphasizes the urgency of responsible actions in our use of resources.
The unique challenge of habitat alteration is its unpredictable nature; species may not reestablish themselves in the altered landscape, paving the way to further declines in biodiversity—taking an already fragile ecosystem to the brink.
Species Extinction
The spectre of species extinction represents one of the most acute consequences of human influence. Climate change alters habitats so drastically that many species no longer fit in, leading to population declines and eventual extinction. This discussion is paramount as it highlights the interconnectedness of climate systems and living organisms.
Notably, the characteristic of species extinction is often irreversible loss. The implications of one species' disappearance ripple across the food web; it’s not just one creature lost, but fragmentations of an entire system. The urgency of addressing this topic can't be understated in our narrative on biology.
Ocean Acidification
Lastly, ocean acidification is a pressing concern tied to climate change, where increased carbon dioxide levels lead to a more acidic ocean environment. Sea creatures, particularly those with shells, such as corals and some plankton, struggle to maintain their structures, leading to further disruptions in marine ecosystems. The relationship between human activity and ocean acidity is clear and sobering.
Future Directions in Biological Research
The landscape of biological research is shifting, with new horizons emerging from the depths of innovation and necessity. As we confront challenges like climate change, loss of biodiversity, and public health crises, understanding and harnessing biological systems has never been more crucial. This section focuses on the forefront of biological research, emphasizing the importance of biotechnology, sustainable practices, and interdisciplinary approaches. Each topic invites its own array of techniques and encourages collaboration across diverse fields, pushing the boundaries of what we know and what we can accomplish.
Biotechnology Innovations
Genetic Engineering
Genetic engineering stands at the forefront of biotechnology. This approach allows scientists to alter an organism's genetic material, offering possibilities from improving crop resilience to developing gene therapies for diseases. The precision of genetic modification is a key characteristic that sets it apart. It's increasingly favored for its ability to make targeted changes, which can lead to significant agricultural and medical advancements.
However, its unique feature also lies in its ethical considerations. While the benefits can be tremendous, discussions about long-term effects and potential risks are equally important. Sometimes, society faces tough questions around genetically modified organisms (GMOs) with regards to safety and environmental impacts.
CRISPR Technology
CRISPR technology represents a significant leap in genetic science. This tool for gene editing allows for precise changes to DNA and is lauded for its efficiency and versatility. CRISPR's ability to target and modify specific genetic sequences has made it a darling in research labs around the globe. It's capturing attention due to its relatively low cost and ease of use compared to older methods.
Nonetheless, like all powerful technologies, CRISPR poses certain risks as well. Unintended consequences of gene editing could have unpredictable effects on ecosystems or human health. Researchers are keen on understanding these potential pitfalls as they continue exploration in this promising field.
Synthetic Biology
Synthetic biology merges biology and engineering, aiming to create new biological parts, devices, and systems. This field seeks to design and construct new biological entities that do not exist in nature. Its key characteristic is its innovative approach to problem-solving, facilitating solutions such as biofuels and pharmaceuticals. The ability to rewrite the genetic code opens exciting avenues for advancements in numerous industries.
However, the implications of synthetic biology can be complex. There are concerns about biosecurity and ethical dilemmas when it comes to creating life forms from scratch. Balancing innovation with precaution is a tightrope walk that researchers in this field must navigate.
Sustainable Practices
Conservation Strategies
Conservation strategies focus on the preservation and restoration of biodiversity. These practices are essential in combating habitat loss and species extinction, making them a critical choice in biological research. The main characteristic of these strategies is their proactive nature, aiming to protect ecosystems before they reach a critical threshold.
One unique feature of conservation efforts is their community involvement, which can create greater awareness about ecological issues. Yet, these strategies can face significant challenges such as funding constraints and public interest variance.
Ecological Restoration
Ecological restoration is the process of assisting the recovery of ecosystems that have been degraded or destroyed. This area of research contributes to biological systems by returning ecosystems to healthy, functioning states. The characteristic of restoration is its focus on long-term resilience and self-sustainability, often combined with community engagement efforts.
The unique aspect of restoration projects is their adaptability to local conditions and cultural contexts. However, these efforts can sometimes be time-consuming and resource-intensive, making them complicated to implement effectively.
Green Technologies
Green technologies aim to reduce environmental impact through innovative solutions like renewable energy sources and waste management systems. Their key characteristic is the goal of sustainability, aligning with the ecological mindset of future research. They are a popular choice among environmentalists and researchers alike due to their potential to create cleaner, healthier habitats.
Despite numerous advantages, the transition to green technologies can involve high initial costs and require significant infrastructure changes. Understanding these barriers can help improve the implementation and acceptance of green technologies in society.
Interdisciplinary Approaches
Systems Biology
Systems biology takes a holistic approach to biological research, emphasizing that complex systems should be studied as integrated wholes rather than isolated components. This perspective allows researchers to see interactions and dynamics between various biological systems. The key characteristic of systems biology is its emphasis on multi-scale modeling, which proves invaluable in studying everything from molecular-level processes to entire ecosystems.
A unique feature is the way it bridges gaps between different scientific disciplines—including biology, computer science, and engineering. Such approaches can potentially result in new discoveries but can also be daunting due to the sheer volume of data involved.
Ecological Modeling
Ecological modeling utilizes mathematical and computational techniques to simulate the interactions in ecosystems. By creating models that predict outcomes based on various factors, researchers can gain insights into the function and health of systems over time. Its primary characteristic is the ability to visualize and analyze complex interactions, which can help inform conservation and management decisions.
A unique strength of ecological modeling lies in its predictive capabilities. However, models are only as good as the assumptions they are built upon. Misjudgment in parameter selection can lead to flawed conclusions, making careful validation crucial.
Integrative Research
Integrative research combines knowledge and methods from various disciplines to tackle complex biological questions. This approach reflects the interconnectedness of biological systems and addresses problems that purely disciplinary research may overlook. The standout feature of integrative research is its capacity to unify disparate findings, drawing from diverse fields to create a cohesive understanding.
Moreover, it encourages collaboration, fostering environments where diverse perspectives can lead to breakthroughs. Nevertheless, aligning goals and methodologies across disciplines can prove challenging and may require additional effort in collaboration.
The continued exploration of these future directions in biological research holds great significance in the face of global challenges. By uniting diverse fields and leveraging innovative technologies, we can create more sustainable solutions for our planet and its inhabitants.
Ultimately, delving into these topics offers a glimpse of the future, underscoring the potential of biological systems research in shaping a more ecologically balanced world.