Understanding Plasma Physics and Fusion Energy Potential
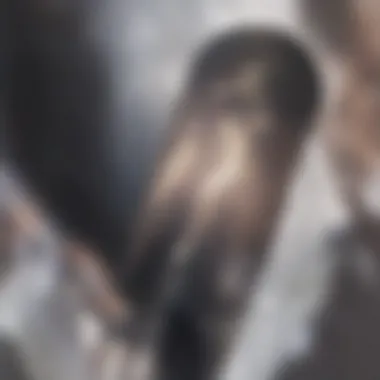
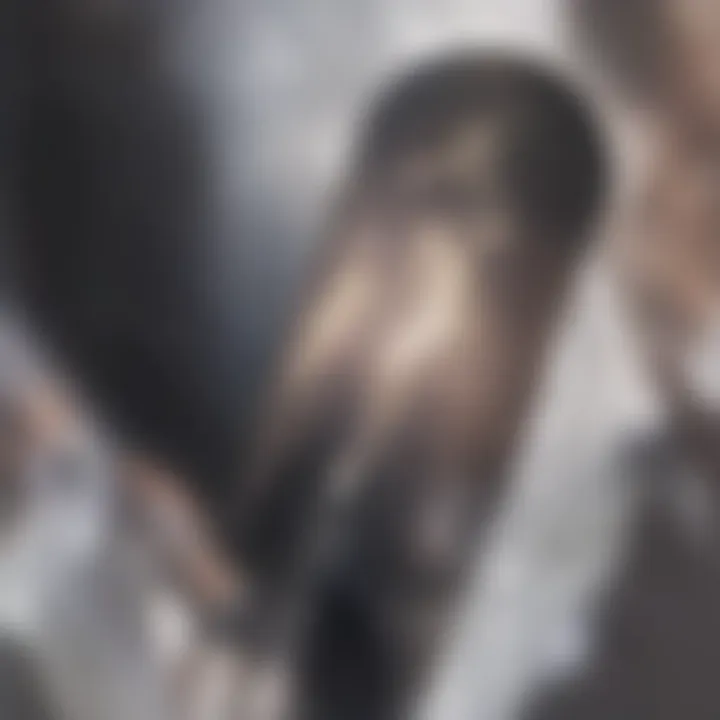
Intro
Plasma physics is a complex and fascinating branch of science that studies ionized gases, referred to as plasmas. This state of matter is prevalent in the universe. Understanding plasma is crucial, especially in the context of fusion energy—an area gaining substantial research attention. Fusion energy promises a sustainable and potent energy source, potentially revolutionizing our approach to energy production.
The relationship between plasma physics and fusion energy cannot be overstated. At high temperatures, plasma can achieve conditions necessary for nuclear fusion. Achieving stable and controlled fusion energy is a challenge that requires deep insights into plasma behavior. This article aims to delve into the fundamental principles of plasma physics, its intricate ties to fusion energy, and the latest advancements in this field.
Research Context
Background Information
The study of plasma physics is built on the understanding of charged particles and electromagnetic fields. Plasma, constituting more than 99% of the visible universe, differs significantly from solids, liquids, and gases. It comprises ions and free electrons and exhibits unique behaviors, such as collective movements and oscillations. Fusion occurs when light nuclei combine to form heavier nuclei, releasing vast amounts of energy in the process. This is the principle powering our sun and stars.
In laboratory settings, researchers have made significant strides in achieving the necessary conditions for fusion, although challenges persist. This study examines how plasma physics informs our understanding of these conditions.
Importance of the Study
The implications of understanding plasma physics within the context of fusion energy are profound. Fusion has the potential to provide a nearly limitless energy source with minimal environmental impact. Unlike fossil fuels, fusion produces minimal greenhouse gases and has less long-lived radioactive waste than traditional nuclear fission methods.
The relevance of studying plasma physics transcends academic interest. As global energy demand escalates and concerns about climate change intensify, exploring sustainable energy sources is critical. This study provides insights that may lead to breakthroughs in fusion technologies, impacting energy policy and development for generations to come.
Future Directions
In moving forward, research in plasma physics must address several key aspects:
- Technological advancements in magnetic confinement and inertial confinement techniques.
- Interdisciplinary collaboration among physicists, engineers, and policymakers.
- Exploration of materials that can withstand extreme conditions within fusion reactors.
"The pursuit of practical fusion energy remains one of the most ambitious goals in modern science. It is not merely a question of physics, but one of sustainability and environmental stewardship."
As researchers continue to grapple with these issues, it is essential not just to advance scientific knowledge but also to communicate findings effectively to a broader audience. This will ensure that policy decisions are informed by the latest scientific insights.
Discussion
Interpretation of Results
Understanding the outcomes of recent experiments in plasma confinement is vital. Researchers have observed phenomena such as plasma stability and confinement duration, which are essential for effective fusion. The implications of these results must be critically analyzed to shape future research agendas.
Comparison with Previous Research
Comparative analyses of historical and current experiments reveal significant progress in plasma physics. Innovations in understanding plasma behavior aid contemporary research efforts. This evolution illustrates the dynamic nature of scientific inquiry in achieving fusion energy.
Foreword to Plasma Physics
The concept of plasma physics is fundamental in understanding the processes that drive fusion energy. Plasma, which is often referred to as the fourth state of matter, encompasses a significant part of the universe and offers potential solutions for sustainable energy production. Its unique characteristics position it as a primary focus in researching avenues such as fusion energy, which could address pressing global energy demands without adverse environmental impacts.
In this section, we will explore what plasma is, its properties, and its wide-ranging applications. Understanding these aspects enriches our insight into plasma physics and its role in the quest for viable fusion energy solutions. The discussion will highlight how fundamental knowledge of plasma can transform theoretical principles into practical technologies.
What is Plasma?
Plasma is a state of matter formed when gas is energized to the point that its atoms lose electrons, resulting in a mixture of ions and electrons. This ionized gas does not have a definite shape or volume, unlike solids, liquids, or gases. Plasma can be created under various conditions, such as heating a gas to very high temperatures or exposing it to strong electromagnetic fields.
Plasma exists naturally in the universe, constituting stars, including our sun. The sun's core, where fusion occurs, serves as a prime example of plasma in action. Understanding plasma is essential for harnessing its properties for energy production.
Properties of Plasma
Plasma has distinct properties that differentiate it from other states of matter:
- Conductivity: Plasma is an excellent conductor of electricity due to the presence of free-moving charged particles.
- Magnetic Field Interactions: Charged particles in plasma respond to magnetic fields, allowing for manipulation of plasma states in controlled environments.
- Temperature Sensitivity: Plasma can exist at extremely high temperatures. For fusion processes, the temperatures needed are in excess of millions of degrees.
These characteristics are vital for the development of fusion reactors, where maintaining stable plasma conditions is essential for successful energy production.
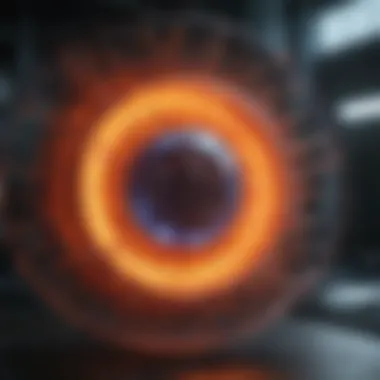
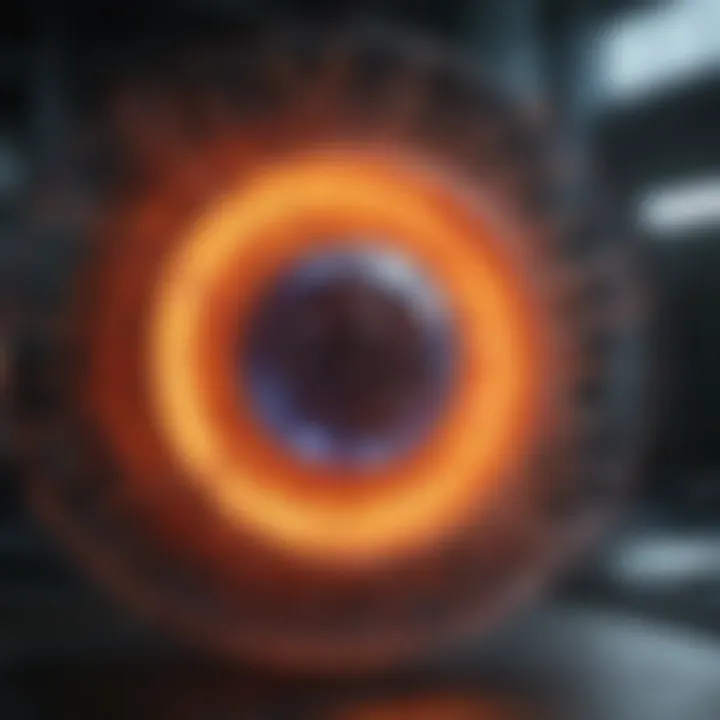
Applications of Plasma Physics
The applications of plasma physics span a wide array of fields beyond fusion energy. Some notable ones include:
- Semiconductor Manufacturing: Plasma processes are used to etch microcircuits and deposit thin films essential for modern electronics.
- Space Propulsion: Electric propulsion systems, such as ion thrusters, utilize plasma for efficient spacecraft maneuvering.
- Medical Technology: Plasma is employed in sterilization processes and dermatological treatments.
In the context of energy production, the applications of plasma physics are integral to advancing fusion research. By understanding the properties and behavior of plasmas, scientists are working towards developing practical fusion reactors that can provide limitless, clean energy.
Foundations of Fusion Energy
Fusion energy represents a pivotal approach to achieving sustainable and nearly limitless power. Understanding the foundations of fusion energy is essential because they lay the groundwork for harnessing plasma physics in practical energy generation. Fusion occurs when light atomic nuclei combine to form heavier nuclei, releasing substantial amounts of energy. This process mirrors what occurs naturally within stars, including our Sun. Exploring the foundational aspects not only highlights the scientific principles at play but also emphasizes the potential benefits of fusion energy, such as minimal environmental impact and the abundance of fuel sources.
The Science of Fusion Reactions
Fusion reactions involve distinct elements, primarily isotopes of hydrogen—deuterium and tritium. When these isotopes are brought together under extreme conditions of heat and pressure, they can overcome their electrostatic repulsion, leading to their fusion. The resultant fusion releases a neutron and a significant amount of energy.
The most common fusion reaction sought after in experiments is:
- Deuterium + Tritium → Helium + Neutron + Energy.
The energy output from fusion reactions is considerable compared to chemical reactions such as combustion. This efficiency propels the ongoing research efforts aimed at reducing the necessary conditions for controlled fusion, thereby making it a viable energy source.
The challenge lies in achieving the required temperature and pressure necessary to initiate these reactions. Scientists aim to replicate the colossal energy of the Sun on a smaller, manageable scale.
To maintain the necessary conditions for sustained fusion, plasma must be confined and controlled. This leads to various methodologies in fusion technology, such as magnetic confinement and inertial confinement, each having its unique mechanisms and objectives.
Contrast with Fission Energy
Fission energy differs fundamentally from fusion. Fission involves splitting heavy atomic nuclei—like uranium or plutonium—into two smaller nuclei, releasing energy in the process. The primary distinctions include:
- Fuel Sources: Fusion relies on abundant isotopes of hydrogen, whereas fission depends on limited nuclear materials.
- Waste Production: Fission generates radioactive waste and has a far greater risk of catastrophic failure. In contrast, fusion produces helium, a harmless gas.
- Safety: Fusion's inherent safety features stem from the reaction's requirements; disruptions lead to an immediate drop in energy production, eliminating the risk of meltdowns.
Understanding these fundamental differences enhances the appreciation of why fusion energy is actively pursued as a cleaner and safer alternative to traditional nuclear fission. The promise of fusion energy continues to inspire researchers around the world, holding the potential for revolutionary developments in energy technology.
"The quest for fusion energy is a journey into the heart of matter, revealing not only how stars shine but how we might create a sustainable future for humanity."
As exploration into fusion energy progresses, continued research in both plasma physics and fusion technology remains crucial for addressing global energy demands while mitigating environmental impact.
The Role of Magnetic Confinement
Magnetic confinement is a pivotal aspect of plasma physics, particularly in the context of achieving practical fusion energy. As fusion reactions require extreme temperatures and conditions, containing the hot plasma presents significant challenges. Magnetic fields are essential because they can effectively stabilize plasma, allowing it to maintain the necessary density and temperature to facilitate fusion processes. This section will explore how magnetic fields contribute to plasma stabilization and the specific systems designed to achieve this goal.
Magnetic Fields in Plasma Stabilization
Magnetic fields play a crucial role in the stabilization of plasma by employing Lorentz forces to govern the motion of charged particles. When a plasma is confined using a magnetic field, the charged particles spiral along the magnetic field lines. The confinement reduces the probability of the plasma coming into contact with the reactor walls, which can be detrimental to sustaining fusion reactions. The effectiveness of magnetic confinement relies heavily on the strength and configuration of the magnetic fields in use.
There are several benefits to using magnetic fields for plasma stabilization:
- Reducing Energy Loss: Magnetic confinement minimizes the energy lost through collisions with reactor materials. By preventing contact with the walls, the plasma can maintain its high temperature, essential for fusion.
- Controlling Instabilities: Plasmas are susceptible to various instabilities that can disrupt confinement. Magnetic fields can suppress these instabilities effectively, ensuring that the plasma remains stable during operation.
- Enabling Long-Term Reactions: Long-duration confinement of plasma is essential for sustaining fusion reactions. Magnetic fields can create distinct confinement regimes, allowing for longer fusion burn times, which is critical for practical energy extraction.
In summary, the role of magnetic fields in plasma stabilization cannot be understated. Their ability to contain high-temperature plasmas while minimizing energy losses makes them a cornerstone of fusion research.
Tokamak Systems Explained
Tokamak systems are one of the most promising approaches in magnetic confinement fusion research. The term "Tokamak" is derived from the Russian acronym that translates to "toroidal chamber with magnetic coils". These systems utilize a combination of two magnetic fields: a toroidal magnetic field, which runs around the doughnut-shaped chamber, and a poloidal magnetic field, which loops around the plasma. This combination helps to contain the plasma more effectively.
A Tokamak operates by inducing a plasma current which generates the poloidal magnetic field. The toroidal field then works to keep the plasma stable and confined in a controlled manner. The geometry and magnetic configuration in a Tokamak system serve to approximate the conditions needed for fusion in stars, harnessing the same principles on Earth.
"The key to fusion power lies in achieving the right conditions to sustain a controlled reaction; this is where the Tokamak design has shown considerable potential."
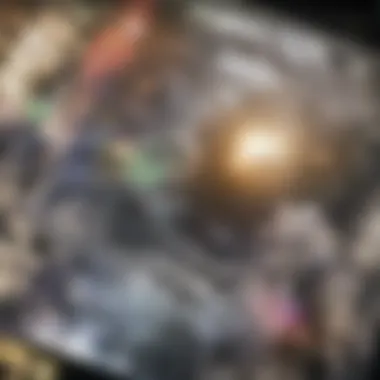
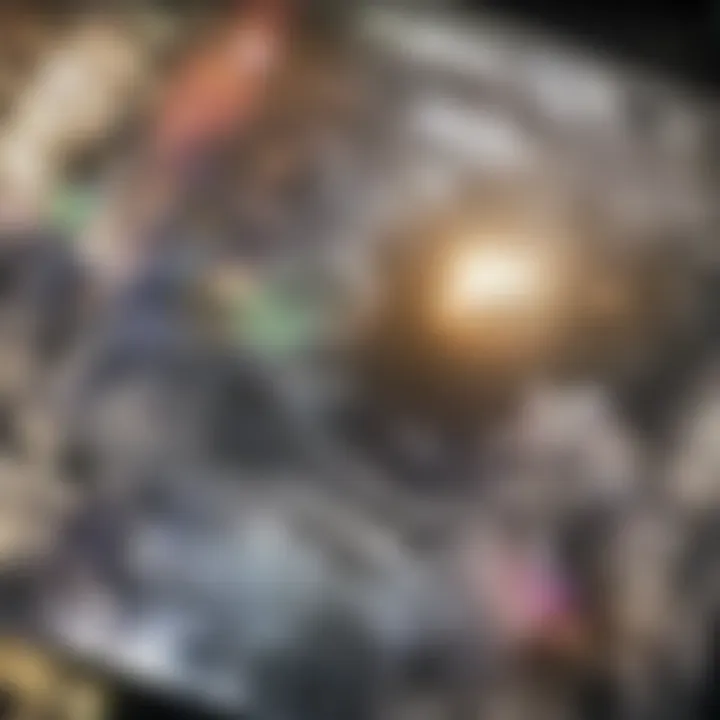
One notable example of a Tokamak is the ITER project. ITER, or the International Thermonuclear Experimental Reactor, is currently under construction in France. It aims to demonstrate the feasibility of fusion as a large-scale and carbon-free source of energy. Through experimental results gathered from the ITER project, researchers hope to not only validate the scientific principles behind fusion energy but also refine the technology needed to make it commercially viable.
Inertial Confinement Fusion
Principles of Inertial Confinement
Inertial confinement fusion (ICF) is a technique used to achieve fusion energy through the application of extreme pressure and temperature. The fundamental principle behind ICF is rather straightforward: tiny fuel pellets composed of deuterium and tritium, isotopes of hydrogen, are compressed rapidly to create the necessary conditions for fusion. These pellets are often the size of a pea and are subjected to a brief but intense pulse of energy.
The compression occurs when a large energy source, most commonly lasers or other forms of energy such as X-rays, targets the fuel pellet. The outer layer of the pellet absorbs this energy, causing it to heat and explode outward. This explosion generates an inward force on the remaining part of the pellet, compressing the inner core to the necessary pressure and temperature to initiate fusion.
The process involves complex physics, as the dynamics of the implosion must be precisely controlled. Understanding the Hydrodynamic Instabilities that can occur during this rapid compression is essential. If not managed, these instabilities can disrupt the symmetry of the implosion, which significantly decreases the efficiency of the process. The success of ICF depends on achieving a condition known as ignition, where the energy produced by the reaction exceeds the energy required to initiate it.
Laser Technology in Fusion Research
Laser technology plays a crucial role in the development of inertial confinement fusion. The most notable facility dedicated to this research is the National Ignition Facility (NIF) located in Livermore, California. NIF utilizes a range of powerful lasers to deliver the required energy to the fuel pellets for compressing them into a fusion state.
The operation of these lasers is intricate. Each laser beam is generated and then amplified before being directed towards the target. The energy is ultimately focused onto the surface of the pellet, producing the necessary shockwaves for compression. One of the key advantages of laser-driven ICF is the precision with which energy can be delivered to the pellet. This precision minimizes the risk of instabilities that could otherwise compromise the fusion process.
Moreover, advancements in laser technology are essential for enhancing the efficiency of ICF. Innovations such as the development of high-repetition-rate lasers and photonic crystal fibers have increased energy output and allowed for more controlled experiments. These advancements facilitate ongoing research and ultimately contribute to the goal of making fusion energy a viable method of power generation.
In summary, inertial confinement fusion represents a promising path toward achieving practical fusion energy. By understanding its principles and leveraging cutting-edge laser technology, researchers aim to overcome the barriers presently hindering fusion as a sustainable energy source.
Current Research Efforts
Research in fusion energy is a dynamic field, driven by the ambition to harness plasma physics for sustainable energy production. The complexities of fusion require innovative approaches. Understanding current research efforts is pivotal, as it highlights global initiatives, technological advancements, and the challenges faced.
International Projects in Fusion Energy
International collaboration is vital for advancing fusion energy research. Notable projects include the International Thermonuclear Experimental Reactor (ITER), which aims to demonstrate the feasibility of fusion as a large-scale energy source. This project engages nations such as Europe, the United States, Russia, China, Japan, and South Korea. By pooling resources and expertise, these countries hope to accelerate discoveries that would be challenging to achieve independently.
Additionally, the SPARC project in the United States focuses on developing a compact fusion pilot plant that might pave the way for commercial fusion energy. These large-scale initiatives have the potential not only to deliver breakthroughs in fusion technology but also to foster international scientific cooperation.
Breakthroughs in Plasma Control
Control of plasma is one of the most significant challenges in fusion research. Recent advancements in plasma confinement and stability through innovative approaches have shown promise. For instance, improved feedback systems and real-time diagnostics are enhancing the ability to stabilize plasma more effectively. Techniques like active control can adjust parameters rapidly to maintain optimal conditions for fusion reactions.
An example of a recent breakthrough is the use of artificial intelligence to monitor and predict plasma behavior. Machine learning algorithms can analyze vast amounts of data from fusion experiments to improve control strategies. This integration of advanced computing with plasma physics marks a critical step toward reliable fusion energy.
Challenges in Achieving Fusion Energy
The pursuit of fusion energy presents significant challenges that need addressing. These challenges can affect the feasibility of fusion as a sustainable energy solution. In understanding plasma physics, it is crucial to recognize the hurdles that researchers and scientists must overcome to harness this potential. The complexities involved in plasma behavior, material limitations, and the economic landscape all play a role in the slow progress toward practical fusion energy.
Technical Limitations
Fusion processes require extreme conditions: high temperatures and pressures. Creating and maintaining these conditions is a technical challenge. Plasma must be heated to millions of degrees for fusion reactions to occur. The methods used include inertial and magnetic confinement. However, maintaining stability in the plasma is difficult.
Additionally, the materials used to contain plasma must withstand intense heat and neutron bombardment. Existing materials face degradation in these environments. Researchers are exploring new materials that can endure such conditions.
"The key to unlocking fusion energy may well reside in advanced materials that resist plasma damage while maintaining structural integrity."
Economic Barriers
Funding for fusion research is another significant challenge. Large-scale projects require substantial investment over many years. Nations and private entities often hesitate to commit funds without immediate returns. The long-term nature of fusion makes it less attractive compared to other energy investments.
Moreover, public perception plays a role. Many may not understand the potential of fusion energy and its long-term benefits. Government policies can influence funding dynamics. A strategic approach to investment often is necessary to foster innovation in fusion technology. Due to these factors, the road to commercially viable fusion is often fraught with obstacles.
Safety and Environmental Concerns
When discussing fusion energy, safety typically comes to the forefront. While fusion is inherently safer than fission, public concerns persist. One area of concern is the handling of tritium, a radioactive isotope utilized in fusion processes. Ensuring safe containment and minimizing environmental impact is critical.
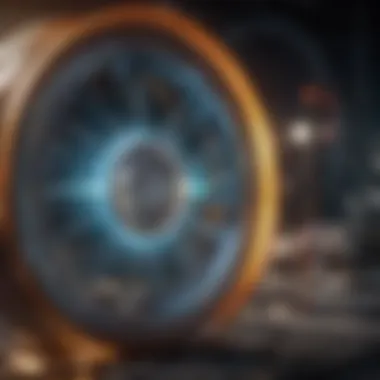
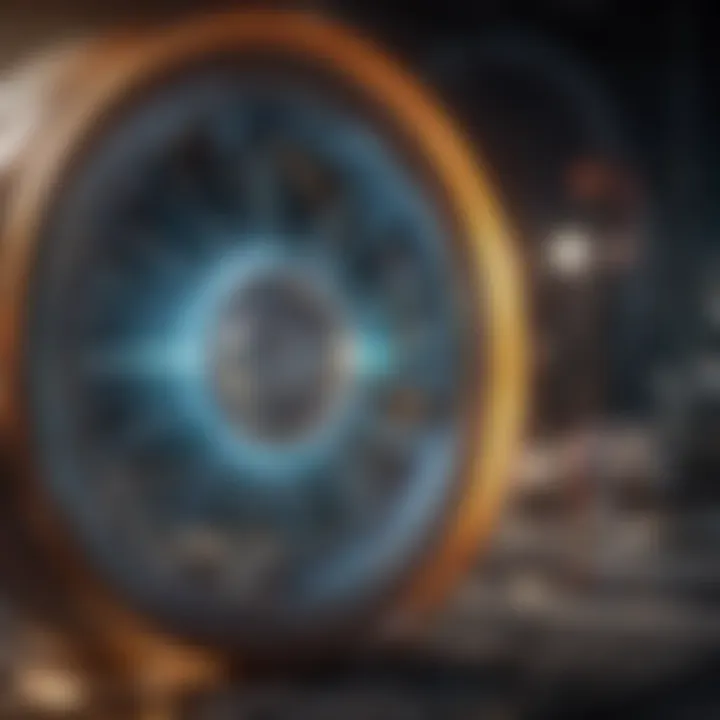
Environmental considerations also include the fusion reactor's footprint and its impact on ecosystems during construction. The production of waste, although far less hazardous than fission, still poses challenges. Adequate strategies must be implemented to address these concerns comprehensively.
In summary, addressing these challenges is vital to progress in fusion energy research. Each aspect—technical, economic, and safety concerns—demands focused effort to unlock the full potential of fusion as a sustainable energy source.
The Impact of Public Policy on Fusion Research
Public policy plays a critical role in shaping the future of fusion energy research. The evolution of technological advancements in this domain is tightly interwoven with decisions made by government officials and agencies. Investment in research funding, collaboration frameworks, and regulatory environments all hinge on current political climates and strategic priorities.
Understanding the nuances of how policy influences fusion research is essential for researchers and stakeholders. A well-defined policy can stimulate innovation, enhance funding opportunities, and facilitate alliances across institutions, both nationally and internationally.
Funding and Investment Trends
Securing funding is pivotal for the advancement of fusion energy technologies. Traditional funding sources include government agencies, private sector investments, and international collaborations.
- Government Funding: Many countries allocate budget to support fusion research. For instance, the U.S. Department of Energy has consistently championed various initiatives that promote scientific inquiry into fusion energy. Such funding can create a foundation for essential research and development by ensuring resources are readily available.
- Private Sector Involvement: Recently, private companies have gained interest in fusion technology. Organizations like Helion Energy and TAE Technologies are investing heavily in alternative fusion approaches. This trend indicates a shift toward market-driven models, potentially accelerating innovations and reducing the time to viable energy solutions.
- International Collaborations: Global projects such as ITER spotlight the importance of sharing knowledge and resources. Countries pooling their efforts magnifies the capabilities of individual nations, facilitating large-scale research that no single country could undertake alone.
Funding trends must also reflect shifts in public policy. Increased governmental support typically encourages further investments at all levels, amplifying the drive toward breakthroughs in fusion technology.
Global Collaborations in Research
Fusion energy research thrives on global collaborations. The quest for sustainable energy solutions transcends borders, making international partnerships vital.
- Shared Expertise: Collaborative efforts enable countries to leverage their distinct strengths. A nation with advanced computational capacity can work together with one that has substantial experimental facilities. This symbiosis creates comprehensive research programs that enrich the field.
- Joint projects: Large-scale international initiatives, such as the ITER project, exemplify how nations can unite for a common goal—achieving practical fusion energy. This initiative represents a multi-national approach combining resources and expertise from Europe, the United States, Russia, Japan, China, and South Korea.
- Standardization and Regulation: Global research collaborations also contribute to establishing standardized practices and regulations. Ensuring safety and environmental concerns are consistently addressed across jurisdictions can prevent haphazard investments and promote more responsible research outcomes.
Policy decisions get to the heart of how these collaborations are structured and sustained. Open channels for dialogue among countries can lay the groundwork for a more coordinated effort in the pursuit of fusion energy.
"The success of fusion energy may depend as much on international collaboration as on scientific breakthroughs."
Ultimately, public policy is not just a backdrop for fusion research. It is a driving force that shapes funding landscapes and enables global collaborations. As the challenges of climate change and energy needs grow more pressing, the nexus between policy, funding, and innovative research becomes increasingly critical.
Future Prospects of Fusion Energy
The domain of fusion energy presents a significant frontier in our quest for sustainable energy sources. As researchers continue to explore the potential of plasma physics and fusion mechanisms, it's clear that the future of this technology could redefine energy production globally. This section examines the innovative technologies that may arise and the crucial environmental considerations associated with fusion energy.
Potential Innovations in Technology
Future advancements in fusion technology are likely to hinge on several key innovations. These innovations are not just technical; they concern materials, engineering, and plasma control methods. Some potential areas of development include:
- Advanced Materials: The next generation of fusion reactors may rely on materials that can withstand extreme temperatures and neutron bombardment. Research into high-temperature superconductors could enhance magnetic confinement systems, leading to more stable plasma conditions.
- Computer Simulations: Enhanced computational models will allow scientists to predict plasma behavior more accurately. This will aid in identifying optimal conditions for sustained fusion reactions, helping to minimize trial-and-error approaches in experimental reactors.
- Laser and Particle Beam Technologies: Innovations in laser systems and particle accelerators may improve inertial confinement fusion techniques. More precise energy delivery mechanisms could lead to better efficiency and higher yield in fusion energy generation.
New Concepts in reactor design, such as spherical tokamaks or liquid metal walls, may also emerge. The combination of these innovations has the potential to make fusion energy more feasible and economical.
Long-term Environmental Considerations
The environmental impact of fusion energy, while often touted as minimal compared to fossil fuels, is complex. Key considerations for the long term include:
- Waste Management: Fusion does produce some radioactive waste, albeit significantly less than fission. Developing strategies for the safe disposal or recycling of materials used in fusion reactors is essential for minimizing long-term environmental risks.
- Impact on Land Use: Large fusion power plants will require considerable land for operation and safety zones. Assessing the ecological impact of site selection is important to ensure that local ecosystems are preserved.
- Energy Transition: As fusion energy development progresses, there will be transitional phases where fossil fuels remain dominant. These periods must be managed carefully to avoid ecological damage and ensure a balanced energy landscape.
"Fusion energy has the potential to provide a nearly limitless energy source, but ethical and environmental considerations must guide its development."
Through thoughtful planning and innovative thinking, fusion may soon become a cornerstone of sustainable energy solutions.
End
The conclusion of this article serves as a culmination of the critical ideas presented throughout the examination of plasma physics and its relationship with fusion energy. Understanding the foundational principles of plasma is paramount. Plasma, as a state of matter, is integral to the mechanics of fusion reactions, which promise a potential solution to global energy challenges.
Summary of Key Findings
In exploring the topic, several key findings emerge:
- Nature of Plasma: Plasma consists of charged particles, which behave in complex ways under varying conditions. This makes plasma both fascinating and challenging to study.
- Fusion Reactions: Fusion has the potential to provide a nearly limitless energy source. However, sustaining the conditions necessary for these reactions remains a primary obstacle.
- Magnetic and Inertial Confinement: Different methods like magnetic confinement and inertial confinement showcase the diverse approaches to achieving controlled fusion. Each method has advantages and constraints, contributing to ongoing research efforts.
- Current Challenges: Technical limitations, economic barriers, and safety concerns need to be addressed. Public policy plays a significant role in shaping the future of fusion energy research and development.
Final Thoughts on Plasma Physics and Fusion Energy
The integration of plasma physics into practical applications in fusion energy could reshape the global energy landscape. This would usher in a new era, where clean, efficient energy can meet the demands of an ever-growing population. Thus, understanding plasma physics is not just an academic exercise; it’s a vital necessity for future energy sustainability.