Understanding Lithium Charge: Mechanisms and Applications
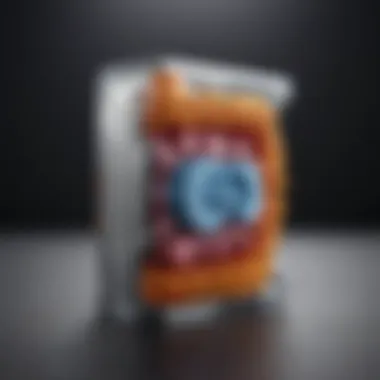
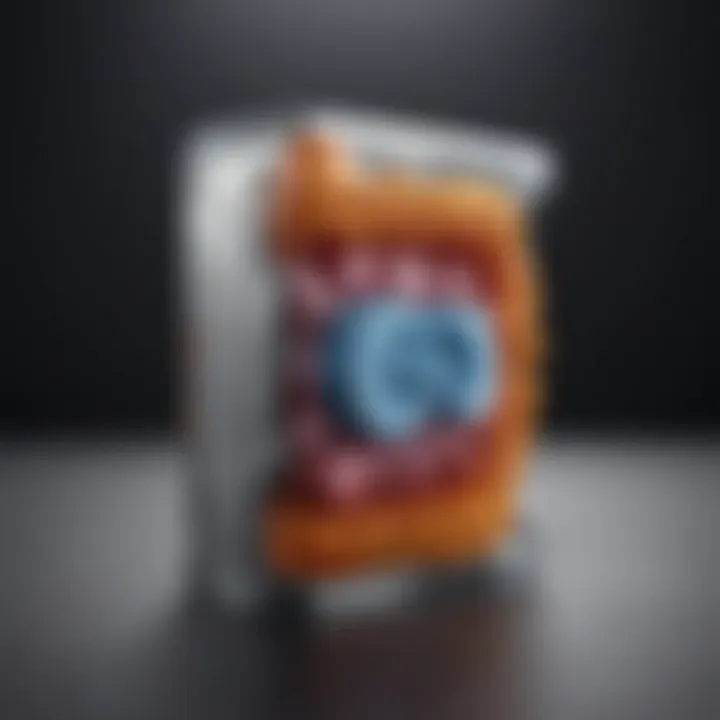
Intro
Lithium charge technology plays a pivotal role in modern energy systems, particularly within the domain of electric vehicles and portable electronic devices. Understanding how lithium charge operates not only enhances our comprehension of its applications but also sheds light on the future of energy storage. This article aims to unpack the intricate mechanisms and functionalities associated with lithium ion cells while exploring their environmental and technological implications.
Research Context
Background Information
The development of lithium-ion batteries has revolutionized the energy landscape over the past few decades. These batteries operate based on the movement of lithium ions between the anode and cathode during charging and discharging cycles. This process is crucial in determining both the efficiency and longevity of the batteries. Traditional rechargeable batteries, such as nickel-cadmium models, have limitations in terms of energy density and cycle life that lithium-based technologies surpass.
Lithium is the lightest metal and has a high electrochemical potential, making it particularly suitable for energy storage. As a result, lithium-ion cells have become ubiquitous in personal electronics, grid storage, and electric vehicles. Understanding the charge mechanisms that govern these systems is essential for improvement and innovation in energy technology.
Importance of the Study
This study is important for several reasons. Firstly, lithium-ion batteries are central to the transition towards more sustainable energy solutions. Their application in electric vehicles can significantly reduce greenhouse gas emissions compared to traditional combustion engines. Secondly, understanding the mechanisms behind lithium charge technology can lead to advancements in battery performance, including increased capacity and faster charging times. Furthermore, by addressing the environmental impacts and sustainability associated with lithium extraction and processing, this research encourages the development of safer and more efficient battery technologies.
In recent years, the rise of alternative battery technologies, such as solid-state batteries and sodium-ion batteries, emphasizes the need for ongoing research in this field. Emerging alternatives could play a significant role in addressing both performance issues and the environmental criticisms related to current lithium systems.
Discussion
Interpretation of Results
The mechanisms involved in lithium ion charging are multifaceted. When a lithium-ion battery is charged, lithium ions are extracted from the cathode and migrate through the electrolyte to the anode, where they are stored. This process occurs reversibly, allowing the battery to cycle multiple times. Understanding this cycle is crucial in tackling issues like lithium plating, which can occur in fast charging scenarios and leads to reduced battery life.
Comparison with Previous Research
Previous research has often focused on enhancing the energy capacity of lithium-ion batteries, but recent studies have increasingly addressed the implications of scaling up lithium production and its environmental impact. For example, articles from Britannica and Wikipedia provide insights on the global lithium market and its exploding demand, which raises concerns about sustainable mining practices and end-of-life battery recycling. Recent advances showcase a diverse array of alternatives being developed alongside lithium technologies, including organic batteries and flow batteries.
A comprehensive examination of lithium charge mechanisms not only builds on existing research but also contributes to a sustainable future in energy consumption.
"Lithium charge technology is not just about improved performance; it also encapsulates the environmental and ethical considerations that need urgent attention in todayβs fast-paced technological advancements."
Understanding the intricate mechanisms of lithium charge technology contributes significantly to this robust academic dialogue, encouraging researchers, educators, and practitioners alike to stay informed and committed to innovation in energy storage.
Preface to Lithium Charge Technology
Lithium charge technology has become a cornerstone of modern energy systems, offering a blend of efficiency and versatility not found in many other energy storage solutions. This technology primarily revolves around lithium-ion batteries, which facilitate the efficient storage and release of electrical energy. Recognizing the importance of lithium charging mechanisms is crucial for understanding contemporary energy demands and technological advancements. Without a grasp of these principles, one cannot fully appreciate the transformative impact lithium technology has on various sectors.
Overview of Lithium-ion Technology
Lithium-ion technology is defined by its efficiency and longevity compared to traditional batteries. These batteries utilize lithium ions as the primary charge carriers, enabling a high energy density while maintaining a lighter weight. The basic operation involves the movement of lithium ions between the anode and cathode. During charging, lithium ions migrate to the anode, while during discharging, they move back to the cathode, thus providing electric power.
The construction of lithium-ion batteries often includes a combination of materials such as graphite for the anode and various metal oxides for the cathode. These materials contribute to the overall functionality, enabling high charge and discharge rates. Additionally, lithium-ion technology supports fast charging capabilities, which are increasingly demanded in applications ranging from consumer electronics to electric vehicles.
Importance in Modern Energy Systems
The relevance of lithium charge technology in today's energy systems cannot be overstated. As global energy demands increase, the need for reliable and scalable energy storage solutions becomes more pressing. Lithium-ion batteries have emerged as a preferred option due to their compactness, efficient charge cycles, and minimal self-discharge rates.
Some key aspects of their importance include:
- Sustainability: Lithium-ion units are pivotal in the shift towards renewable energy. They facilitate the storage of energy generated from sources like solar and wind, allowing for energy usage when it is most needed.
- Transportation Revolution: In the automotive sector, electric vehicles rely heavily on lithium-ion technology to enhance performance and reduce carbon emissions.
- Portable Technology: The proliferation of smartphones, laptops, and other portable devices can be largely attributed to the advances in lithium-ion battery technology, offering users greater convenience without sacrificing mobility.
"Lithium-ion technology is not just a battery; it represents a shift in how we think about energy storage and consumption in our daily lives."
Overall, understanding lithium charge technology, particularly lithium-ion systems, is essential for anyone involved in energy, technology, or environmental studies. It opens up discussions on efficiency, innovation, and the future of energy solutions.
Fundamental Principles of Lithium Charging
Lithium charging technology is crucial for advancements in various energy systems. Understanding the fundamental principles of lithium charging offers insights into how these systems operate effectively. This knowledge is vital, as it influences the design and efficiency of lithium-ion batteries, which have become prominent power sources in today's technology-driven world.
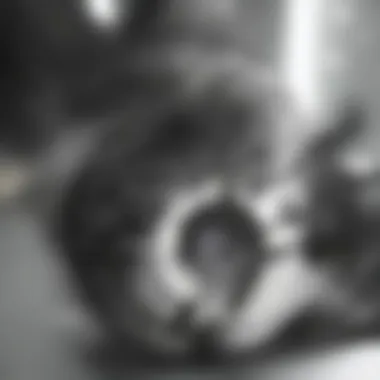
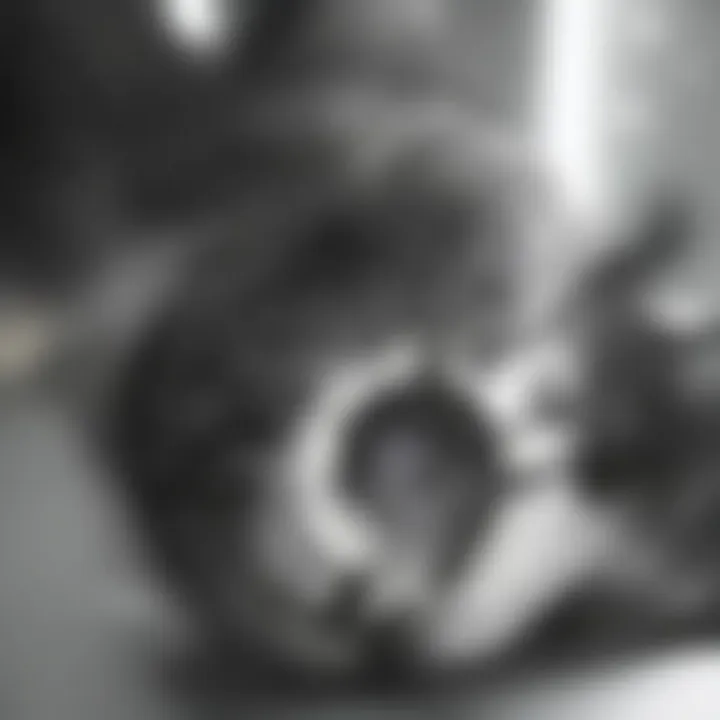
Basic Electrochemical Concepts
To grasp lithium charging, one must first understand the basic electrochemical concepts involved. At the heart of any battery is an electrochemical cell, consisting of two electrodes: an anode and a cathode, separated by an electrolyte. During charging, lithium ions move from the cathode to the anode through the electrolyte, storing energy. Conversely, during discharging, these ions move back to the cathode, releasing energy. This movement of ions is facilitated by the oxidation and reduction reactions that happen at both electrodes.
Key components of electrochemical cells include:
- Electrodes: These are materials that conduct electricity. The anode is typically made of graphite, while the cathode often consists of lithium cobalt oxide or lithium iron phosphate.
- Electrolyte: This medium allows for the transfer of lithium ions. Liquid, gel, and solid electrolytes are common in various lithium battery designs.
- Separator: This part keeps the electrodes apart, preventing short circuits while allowing the passage of lithium ions.
Understanding these components and their roles helps clarify how lithium-ion batteries function and why they are effective. The efficiency with which lithium ions can move through the battery system dictates the performance of the battery, influencing charging times and energy capacity.
Role of Lithium Ions in Energy Storage
Lithium ions play a pivotal role in energy storage solutions. Their unique properties make them suitable for high-efficiency battery applications. Lithium ions are lightweight, possess a high electrochemical potential, and can intercalate into various host materials without significant volumetric change. These characteristics contribute to the overall energy density of lithium-ion batteries, making them effective for applications requiring a compact and powerful source of energy.
- Energy Density: Lithium-ion batteries have a higher energy density than other rechargeable batteries, allowing them to store more energy in less space.
- Cycle Stability: Lithium ions can endure many charge and discharge cycles without degrading. This longevity is essential for applications in consumer electronics and vehicles.
- Lower Self-Discharge Rate: Lithium batteries retain their charge for longer when not in use, which enhances their viability in energy storage systems.
It is important to note that the behavior of lithium ions during charge cycles can affect the overall battery performance. Factors such as temperature, charge rates, and the choice of materials influence the efficiency of lithium-ion movement, impacting both the durability and safety of the batteries.
"Understanding the fundamental principles behind lithium charging technology is essential for optimizing energy storage solutions and advancing the field of battery technologies."
Mechanisms of Lithium Charging
The mechanisms involved in lithium charging are fundamental to understanding how lithium-ion technology functions. This subject is critical for several reasons. First, knowing how lithium batteries charge allows us to optimize their use in applications from handheld devices to electric vehicles. It emphasizes the relationship between the charge cycles and battery lifespan, which benefits users by helping to extend performance over time. Additionally, understanding the mechanisms can inform researchers and developers about improving overall capacity and efficiency, leading to more sustainable energy systems.
Charge Cycle Explained
The charge cycle of a lithium battery consists of two primary phases: charging and discharging. During the charging phase, lithium ions move from the positive electrode, typically made of lithium cobalt oxide, into the negative electrode, commonly made of graphite. This transfer occurs when an external power source provides energy, allowing electrons to flow through the circuit.
Once the battery is connected to a power source, the following processes take place:
- Lithium Ion Movement: Lithium ions migrate through the electrolyte from the cathode to the anode.
- Electron Flow: Electrons move through the external circuit from the cathode to the anode, creating electrical energy.
- Energy Storage: The energy from the electrons provides the necessary force for the lithium ions to embed themselves within the anode material.
As the battery discharges, the process reverses. Lithium ions move back to the positive electrode, releasing energy that can be utilized to power devices. This cyclical process is crucial for the functionality of lithium-ion batteries, making it essential to consider factors influencing charge efficiency.
"The efficiency of a lithium battery during its charge cycle significantly impacts its overall performance and longevity."
Factors Influencing Charge Efficiency
Several factors can affect the efficiency of lithium charging. Understanding these aspects is vital for optimizing battery performance and enhancing longevity. Key factors include:
- Temperature: Charging at extreme temperatures, either high or low, can hinder performance. Optimal charging occurs within a specific temperature range, often between 20 and 25 degrees Celsius.
- Charge Rate: The speed at which a battery is charged, expressed in C-rate, influences efficiency. A higher charge rate may lead to quicker charging but can generate excess heat, potentially damaging the battery.
- Electrode Materials: The type of material used for electrodes affects ionic conductivity and overall charge efficiency. Materials with high conductivity enhance ion movement and improve efficiency.
- State of Charge (SoC): The battery's state of charge can impact efficiency, especially in the lower and upper charge levels. Charging closer to full capacity can decrease the charge rate due to protective circuitry.
In sum, the mechanisms of lithium charging are essential to grasping the intricacies of lithium-ion batteries. Understanding the charge cycle and the factors influencing efficiency provides insights critical for advancing battery technologies and their applications.
Technical Aspects of Lithium Charge Systems
The technical aspects of lithium charge systems are fundamental to comprehending how electricity storage and transfer operate within various applications, including consumer electronics and electric vehicles. Understanding these elements is essential for optimizing performance, enhancing efficiency, and ensuring safety in lithium-ion batteries, which are pivotal in today's technology landscape. These systems define how well batteries perform, their lifespan, and how effectively they can be integrated into broader energy solutions.
Types of Lithium Batteries
Lithium batteries are not one-size-fits-all; they come in various shapes and technologies tailored for specific uses. Their design influences energy density, thermal management, and overall application suitability.
Prismatic Cells
Prismatic cells are rectangular in shape, allowing for efficient use of space. This design enables modular battery packs that can be easily scaled and configured for numerous applications, notably in electric vehicles and energy storage systems.
A key characteristic of prismatic cells is their flat structure, which aids in excellent thermal management. This is important because overheating can lead to performance degradation or safety issues. Moreover, their compact size can provide distinct advantages for manufacturers seeking to minimize weight while maximizing energy capacity.
However, prismatic cells have their limitations, such as complexities in manufacturing processes. As a result, their production costs can be higher compared to other types.
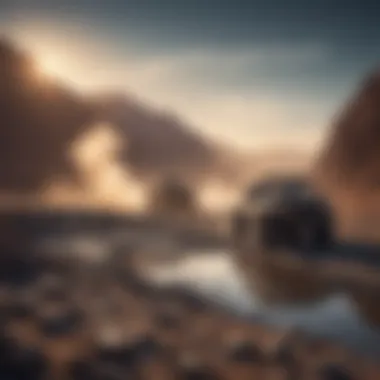
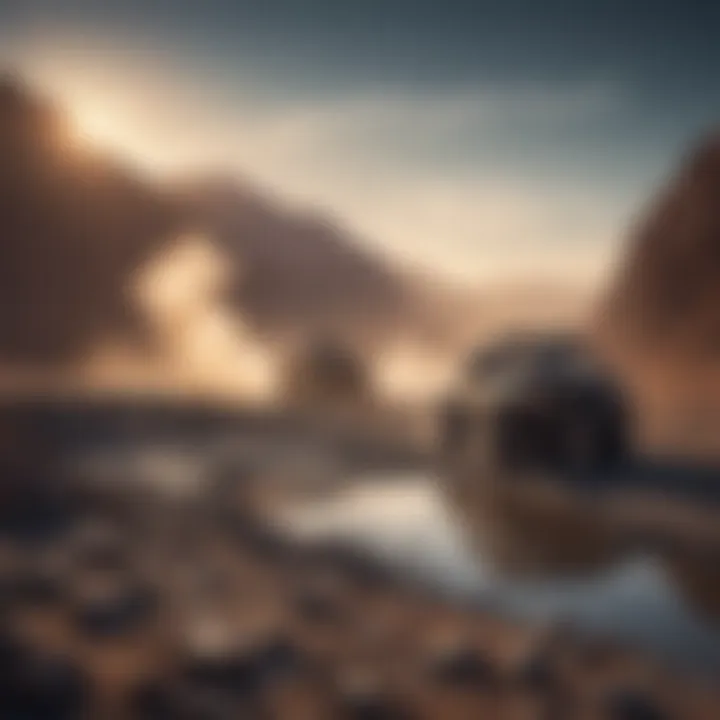
Cylindrical Cells
Cylindrical cells are well-known for their robustness and high energy density. They are often used in consumer electronics as they offer a reliable power source with good cycle stability. This makes them an appealing choice for devices like laptops and smartphones, where space and weight are vital considerations.
The round shape of cylindrical cells contributes to their exceptional structural integrity. They can withstand physical stress better than flat cells. Additionally, they are less prone to thermal runaway due to better heat dissipation properties.
However, cylindrical cells can waste space in battery packs due to their shape, often requiring more complex arrangements, which can affect overall design efficiency.
Pouch Cells
Pouch cells are designed in flexible, lightweight pouches, combining a high specific energy with a significantly reduced weight. This design is particularly beneficial in applications where weight is a critical factor, such as drones and electric vehicles.
A notable characteristic of pouch cells is their ability to be manufactured in various sizes and shapes, making them highly adaptable to different designs. Their high energy-to-weight ratio makes them very popular for modern applications, where every gram counts.
On the downside, pouch cells generally offer less structural integrity compared to cylindrical and prismatic cells. They may require extra protective casing, which can add to costs and weight.
Battery Management Systems
Battery management systems (BMS) play a crucial role in the operation of lithium charge systems. These systems manage charging and discharging processes, ensuring maximum performance and lifespan of batteries. The BMS monitors each cell's voltage and temperature, providing critical data that helps avoid conditions that can lead to overcharging or overheating.
Additionally, a well-designed BMS can enhance the overall safety of lithium battery systems by preventing thermal runaway and ensuring that the battery operates within safe limits. As technology advances, BMS are becoming more sophisticated, featuring capabilities for wireless communication, data logging, and predictive maintenance.
"Battery management systems are not just an add-on; they are essential for safe and efficient lithium charge systems."
Applications of Lithium Charging Technology
Lithium charging technology plays a crucial role in various sectors due to its efficiency and energy density. It not only provides power but also enables advancements that are transforming industries. Understanding its applications allows for insights into how lithium systems influence our daily lives and future technologies. This section will explore three main areas where lithium charging technology is integral: consumer electronics, electric vehicles, and renewable energy storage.
Consumer Electronics
Consumer electronics represent one of the largest markets for lithium-ion batteries. These devices include smartphones, laptops, and tablets. Lithium batteries are favored in this sector for their light weight and high capacity. The ability to recharge quickly makes them perfect for gadgets that require constant power.
- Longevity: Most lithium batteries have a longer lifespan compared to alternative chemical batteries, leading to lower replacement costs over time.
- Performance: Devices perform better because lithium can deliver a stable voltage and supports high energy output.
- Portability: As electronics become smaller, the need for compact energy sources increases. Lithium batteries satisfy this requirement effectively.
The increasing demand for portability in consumer gadgets is a driving aspect of lithium battery development. Companies continually strive to enhance capacity and reduce charging times to meet consumer expectations.
Electric Vehicles
Electric vehicles (EVs) are another major application of lithium charging technology. This field has gained substantial traction, paralleling global efforts to reduce greenhouse gas emissions. Lithium-ion batteries excel in delivering the power needed for vehicle propulsion.
- Range: Lithium batteries provide the range needed to make electric vehicles appealing. With new technologies, manufacturers are achieving greater distances on a single charge.
- Efficiency: The efficiency of lithium-ion batteries means that less energy is wasted during use, making electric vehicles more economical compared to traditional gas-powered options.
- Infrastructure: The growth of charging stations improves accessibility, further driving EV adoption.
As automakers pivot towards electric vehicles, the demand for advanced lithium batteries will likely increase, fostering innovations in battery technology and design.
Renewable Energy Storage
The transition to renewable energy sources, such as solar and wind, underscores the importance of lithium charging technology. Lithium-ion batteries serve as a reliable storage solution that stabilizes the inherent variability of these energy sources.
- Energy Storage: Battery systems store excess energy generated during peak hours, which can be used when production decreases.
- Grid Stability: By providing energy when needed, lithium batteries help balance supply and demand on the grid, contributing to a more stable energy system.
- Integration: Modern homes utilize lithium batteries in conjunction with solar systems to maximize energy efficiency and reduce reliance on traditional power sources.
In sum, the applications of lithium charging technology span critical industries. From enhancing consumer devices to powering electric vehicles and supporting renewable energy, lithium technology not only fulfills current demands but also paves the way for future advancements. Its importance will continue to grow as our society moves towards more sustainable energy solutions.
"As the world pushes for cleaner energy solutions, lithium charging technology will remain at the forefront of innovation and application."
Understanding these applications allows stakeholders to appreciate the pivotal role lithium charging technology holds in shaping our modern world.
Challenges in Lithium Charge Technology
The rapid advancement of lithium charge technology has brought significant benefits in energy storage and application. However, this progress is accompanied by several real challenges that must be addressed. Understanding these challenges is crucial for researchers and professionals in the field to formulate effective solutions and ensure the sustainable development of lithium-based energy systems.
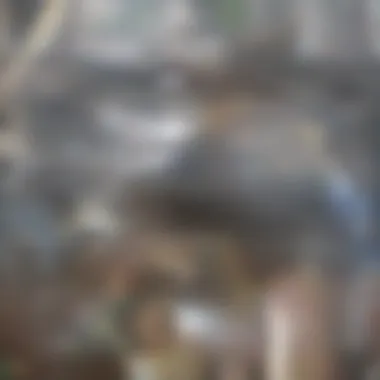
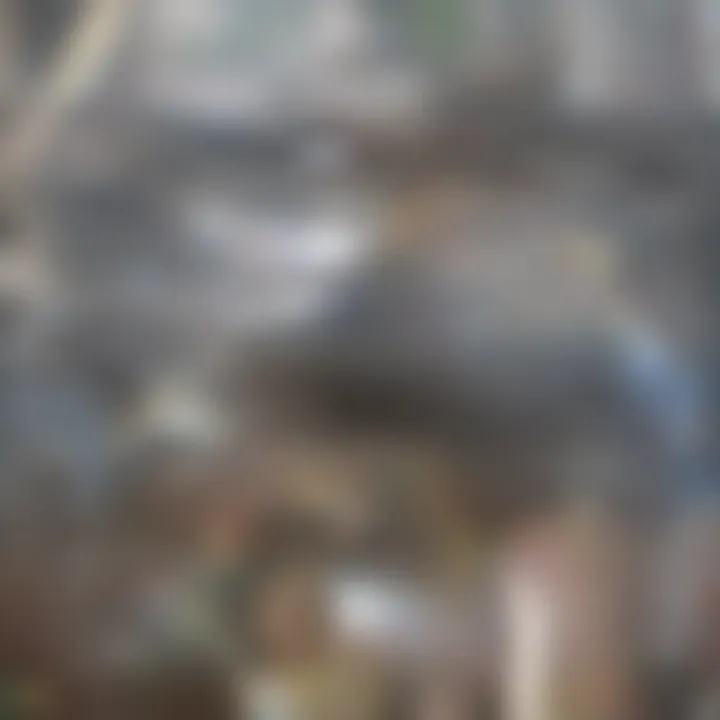
Environmental Concerns of Lithium Mining
Lithium mining is essential for the production of lithium-ion batteries, however, it raises serious environmental concerns. The extraction process can lead to land degradation, water scarcity, and pollution. Large-scale mining operations often require significant amounts of water, which can deplete local sources and affect nearby ecosystems. Furthermore, harmful chemicals used in mining can contaminate soil and water supply.
Another aspect is the carbon footprint associated with lithium extraction. Reducing greenhouse gas emissions has become a priority in today's energy strategy. The methods currently employed for lithium extraction often do not align with these goals.
Additionally, improper waste management from mining sites can result in long-lasting environmental damage. Addressing these concerns requires better regulation, innovative extraction techniques, and a concerted effort to minimize the environmental impact of mining practices.
Safety Issues Related to Lithium Batteries
Safety is a prominent concern for lithium batteries. These batteries can present risks such as overheating, fires, and even explosions. The composition of lithium batteries makes them sensitive to temperature changes and physical stress. Several incidents have highlighted these hazards, especially in consumer electronics and electric vehicles.
To mitigate these risks, manufacturers must prioritize the development of robust Battery Management Systems. These systems play a key role in monitoring battery health, ensuring safe operation, and preventing overcharging. The integration of advanced technologies, such as thermal management and voltage regulation, can enhance the safety of lithium batteries.
Regulations and guidelines regarding lithium battery production and handling also need to evolve continuously. Ensuring that consumers and manufacturers adhere to these standards is vital for improving overall safety.
Future Directions in Lithium Charge Development
Exploring the future of lithium charge technology is essential for understanding how energy storage will evolve. This section delves into innovations and alternatives that could reshape the lithium landscape. It reconsiders the existing paradigms to enhance performance, efficiency, and sustainability. The advancements in this field can significantly impact various sectors, from consumer electronics to large-scale energy systems. Therefore, it's not merely about technology progression but also addressing broader societal and environmental implications.
Innovations in Battery Technology
Battery technology is at the forefront of lithium charge advancements. Researchers focus on improving energy density and shortening charging times. One aspect under investigation is the integration of advanced materials that enhance performance. For instance, new cathode materials such as lithium-rich layered oxides promise to increase energy capacity. Additionally, improvements in electrolyte formulations enhance ionic conductivity. These innovations make batteries not only more efficient but also increasingly capable of meeting the demands of future technologies.
Moreover, smart battery management systems are gaining interest. These systems can predict usage patterns, manage charge cycles intelligently, and prolong battery life. Such advancements indicate a trend toward more sophisticated solutions, making lithium batteries more reliable and user-friendly.
Emerging Alternatives to Lithium
The movement towards sustainable energy includes exploring alternatives to lithium-ion technology. Various materials present themselves as viable options, each with unique characteristics and potential benefits.
Sodium Ion Technologies
Sodium-ion technologies stand out due to their abundance and low cost. Sodium, being the most abundant alkali metal, presents a compelling case for large-scale energy storage solutions.
One key characteristic of sodium-ion batteries is their similar operating principle to lithium-ion batteries. However, sodium ions are larger, resulting in lower energy density. Despite this, sodium-ion batteries can assert some advantages, such as reduced environmental impact from material sourcing. Furthermore, their performance in lower temperatures tends to be advantageous for specific applications.
These batteries hold promise for grid storage, particularly where cost efficiency outweighs the need for high energy density. As a strategic move to diversify battery technologies, sodium-ion systems could play a significant role in the overall landscape.
Solid-State Batteries
Solid-state batteries introduce another innovative approach. They utilize solid electrolytes instead of liquid ones, which mitigates several safety concerns. Their higher energy density is a noteworthy feature. These batteries have the potential to store more energy within the same volume, making them attractive for electric vehicles and portable devices.
A distinct advantage of solid-state batteries is their improved safety profile. They reduce the risk of leakage and flammability associated with liquid electrolytes. However, challenges in manufacturing scale and costs remain. This technology is still in development, yet it holds promise for transforming energy storage applications.
Other Advanced Materials
Other advanced materials are also being researched. Graphene, for example, shows great potential due to its exceptional electrical conductivity and mechanical strength. Utilizing graphene in electrodes could enhance the performance of batteries significantly.
Furthermore, lithium-sulfur batteries are capturing attention for their theoretical energy density advantages over conventional lithium-ion batteries. They could potentially lower the material costs and offer significant weight savings for applications like aerospace. However, challenges with cycle stability and efficiency need to be addressed before they can become mainstream.
The End
The conclusion of this article plays a vital role in reinforcing the overarching themes discussed throughout the sections on lithium charging technology. This is where synthesis occurs. It is not merely a recap; instead, it unveils the significance and implications derived from the discussions on fundamental principles, mechanisms, applications, and future directions in lithium charge technology.
Summary of Key Findings
In the previous sections, we explored various aspects of lithium charge technology. Key findings include:
- Electrochemical Foundations: At the heart of lithium-ion cells lies the movement of lithium ions, which plays a crucial role in energy storage and release during charging cycles.
- Battery Types: Different types of lithium batteries such as prismatic cells, cylindrical cells, and pouch cells each have unique characteristics and applications, catering to different energy storage needs.
- Applications Across Sectors: Lithium charge technology finds application in consumer electronics, electric vehicles, and renewable energy storage, indicating its pervasive role in modern life.
- Challenges Faced: Environmental concerns regarding lithium mining and safety issues related to battery usage raise significant considerations for sustainability.
- Future Innovations: Emerging alternatives, such as sodium-ion technologies and solid-state batteries, suggest a landscape of ongoing innovation that will address some of the challenges faced by current lithium technology.
The Future of Energy Storage Solutions
Considering the rapid advancements in technology and increasing demand for efficient energy solutions, the future of energy storage is poised to evolve significantly. Innovations are likely to focus on several key areas:
- Next-Generation Batteries: Research is underway to develop batteries with improved energy densities, enhanced safety, and lower environmental impact. Advances in solid-state battery technology could provide robust solutions.
- Alternative Materials: Exploring sodium-ion and other advanced materials will be crucial in mitigating the reliance on lithium, and in enhancing battery sustainability.
- Integration with Renewable Sources: As renewable energy sources grow, integrating energy storage with solar and wind technologies will be key in stabilizing energy supply and optimizing usage.