Understanding Electric Car Components: A Detailed Guide
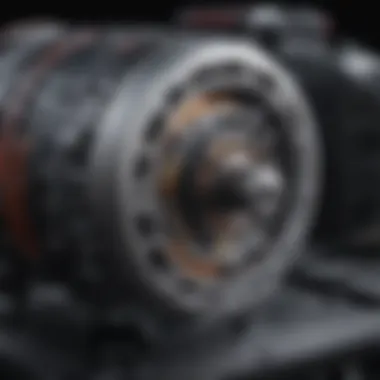
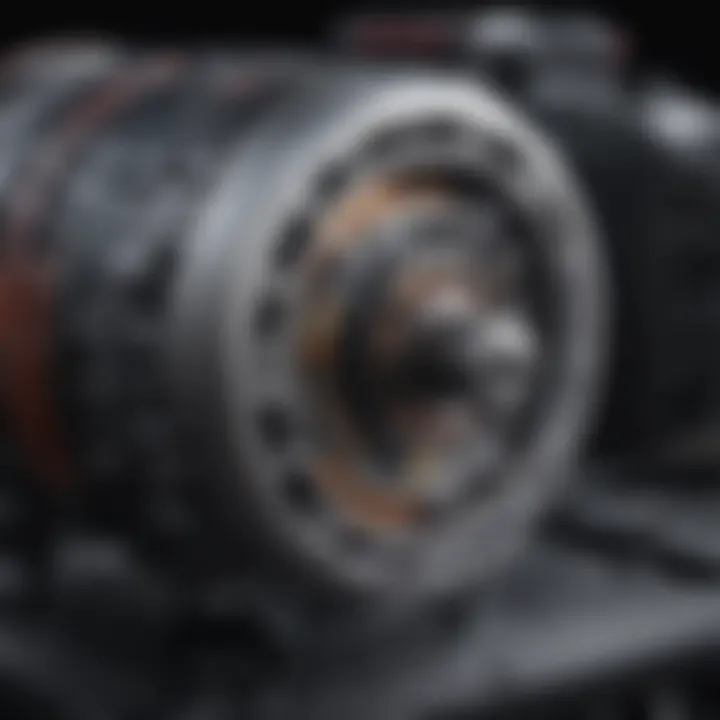
Intro
In this article, we will examine the various components that make up electric vehicles. We will spotlight critical elements such as the electric motor, battery management system, and regenerative braking. Assessing these components aids in grasping how they contribute to the overall efficiency and effectiveness of electric cars.
Research Context
Background Information
Electric vehicles have been on the market for decades now, but only recently have they gained significant traction. The innovation in battery technology and electric drivetrains has positioned EVs as viable competitors to traditional internal combustion engines.
To explore the electric vehicle's functionality, one must consider the advancements in design and engineering that have occurred in recent years. This includes looking into components like lithium-ion batteries and electric drivetrains, which are central to the electric vehicle's success.
Importance of the Study
Understanding the components of electric vehicles is crucial for several reasons. Firstly, it equips industry professionals with the insight needed to innovate further in this field. Secondly, it fosters academic research that can lead to new technologies and methods. Lastly, for consumers, having knowledge about these components can lead to more informed purchasing decisions.
As this technology progresses, staying aware of its components will ensure that individuals and organizations adapt to evolving market trends effectively.
Prelims to Electric Vehicles
The significance of electric vehicles (EVs) in today's automotive landscape cannot be overstated. With growing concerns regarding fossil fuel dependency and climate change, EVs offer a sustainable alternative that aligns with modern environmental goals. This section aims to provide a foundational understanding of electric vehicles, highlighting their essential definitions, historical context, and the evolution that has shaped them into what we see today.
Definition and Overview
Electric vehicles are defined as automobiles that are propelled by an electric motor instead of an internal combustion engine (ICE). They use electricity stored in batteries to power the vehicle, making them distinct from traditional gasoline or diesel-powered cars. There are several types of electric vehicles, including battery electric vehicles (BEVs), plug-in hybrid electric vehicles (PHEVs), and hybrid electric vehicles (HEVs). Each category has unique characteristics that cater to varying consumer needs.
The importance of electric vehicles lies not just in their operational differences but also in their potential impact on energy consumption and emissions. EVs contribute to reduced greenhouse gas emissions, promoting cleaner air and lowering the carbon footprint associated with transportation. In addition, electric vehicles can utilize renewable energy sources, further enhancing their environmental benefits.
Historical Development
The development of electric vehicles can be traced back to the early 19th century. The first practical electric cars appeared in the 1830s, constructed by pioneers like Robert Anderson. However, it was not until the late 19th and early 20th centuries that electric vehicles gained substantial popularity. In fact, in the early 1900s, EVs were among the most favored vehicles in urban areas, due to their quiet operation and ease of use.
Factors contributing to their decline included the rise of gasoline-powered vehicles, which became more affordable and offered greater range and convenience. Post World War II, the global automotive landscape focused heavily on internal combustion engines.
However, concerns about energy security, air pollution, and climate change have fueled a resurgence in interest toward electric vehicles since the late 20th century. Technological advancements in batteries, electric motors, and charging infrastructure have made EVs a practical choice for many consumers, rekindling interest and investment in their development.
Understanding the foundations of electric vehicles is crucial in grasping their integral components and technologies that follow. The modern revolution in EV technology continues to shape the automotive industry, encouraging researchers and professionals to explore these components further.
Electric Motor
The electric motor is a central component of electric vehicles. It provides the necessary power to propel the vehicle efficiently. Unlike conventional internal combustion engines, electric motors convert electrical energy directly into mechanical energy. This method of energy transformation is advantageous due to its high efficiency and lower emissions. Understanding the types of electric motors is crucial as each type has its strengths and weaknesses, making them suitable for different applications in electric vehicles.
Types of Electric Motors
AC Motors
AC motors are widely used in electric vehicles for their robustness and efficiency. They operate using alternating current, which allows them to maintain consistent power and speed. A key characteristic of AC motors is their ability to generate high torque at various speeds, which is essential for vehicle performance. Their beneficial nature lies in their simplicity and durability, often requiring less maintenance compared to other motor types. Additionally, AC motors can also be lighter, which is beneficial for vehicle design. However, one disadvantage is that they require a more complex control system, which can add to overall costs.
Motors
DC motors offer a different approach by utilizing direct current. One specific aspect is that they provide excellent starting torque, making them favorable for applications requiring strong acceleration from a standstill. A major characteristic of DC motors is their straightforward control mechanism, making them easier to manage in many instances. This simplicity makes them a popular choice for smaller electric vehicles and applications where compactness is important. While DC motors can be simpler, they tend to wear out faster due to brush friction, which can lead to maintenance issues over time.
Brushless Motors
Brushless motors are an advanced type of DC motor that eliminates the need for brushes, leading to enhanced efficiency and reliability. They have become increasingly common in electric vehicles. The unique feature of brushless motors is their higher efficiency and longer lifespan, as they reduce wear and tear associated with traditional motors. Additionally, they are known for quieter operation, which is an appealing trait for electric vehicles. However, the complexity of their control systems can be a disadvantage, requiring additional components and potentially increasing costs.
Functionality and Performance
The functionality and performance of electric motors directly affect the overall driving experience of electric vehicles. This section explores how these motors convert electrical energy into motion and the subsequent impact on driving dynamics. Performance metrics would include acceleration capabilities, efficiency ratings, and thermal management. The choice of motor also influences energy consumption and range, which are critical factors for users. Understanding these elements helps in appreciating how different motors contribute to the electric vehicle's effectiveness.
Battery Technologies
Battery technologies are crucial for the performance and efficiency of electric vehicles. They directly influence the range, charging speed, and overall user experience of EVs. As the industry transitions towards more sustainable transport, the advancement in battery technology stands at the forefront. Understanding these technologies is essential for anyone involved in the electric vehicle market, be it a researcher, student, or industry professional.
Lithium-ion Batteries
Lithium-ion batteries are the most prominent type in electric vehicles today. They offer several advantages, including a high energy density, which allows for more energy storage in a compact size. This efficiency plays a significant role when considering the weight and space constraints of EV design.
The lithium-ion chemistry permits faster charging compared to other battery types. This is important for users who rely on limited time frames for charging, making these batteries practical for everyday use. However, they do have limitations, such as thermal runaway risks and degradation over time, which hinder their long-term performance. In terms of cost, lithium-ion technology continues to evolve, with prices decreasing substantially over recent years. Therefore, they strike a balance between performance and affordability, making them a staple in modern EV production.
Solid State Batteries
Solid state batteries represent the next generation of battery technology for electric vehicles. Unlike lithium-ion batteries, solid state designs use a solid electrolyte instead of a liquid one, promising higher energy densities and improved safety. The absence of flammable liquid electrolytes reduces overheating risks, making solid state batteries less prone to fires.
Notably, they can potentially offer larger capacities in smaller packages. This innovation could enhance vehicle design flexibility. However, there are challenges that need addressing, such as manufacturing processes and costs of materials. Research efforts ongoing to overcome these hurdles, positioning solid state batteries as a significant future player in the EV landscape.
Battery Capacity and Range
Battery capacity refers to the amount of electric energy a battery can store, often measured in kilowatt-hours (kWh). A higher capacity indicates longer driving ranges, a critical factor for users who travel longer distances. The range an electric vehicle can achieve on a full charge influences purchase decisions significantly. Currently, most EVs equip batteries ranging from around 30 kWh to over 100 kWh.
Factors affecting range also include driving style, terrain, and temperature. Understanding these can help optimize the use of electric vehicles. Additionally, ongoing advancements in battery technologies contribute to a gradual increase in range capabilities, addressing user concerns about charging infrastructure and travel plans.
"Battery innovation continues to transform electric vehicles, bringing us closer to a sustainable future in transportation."
As battery technology progresses, its role in the electric vehicle ecosystem becomes even more vital, warranting further research and understanding among stakeholders.
Battery Management System
Battery Management Systems (BMS) play a critical role in the functionality and longevity of electric vehicles. This technology is essential for optimizing battery performance, ensuring safety, and extending battery life. Understanding BMS is vital for comprehending how electric vehicles operate efficiently and sustainably.
Importance of BMS
The primary function of a BMS is to monitor and manage the various elements of battery packs. Without a proper BMS, batteries can become unstable, leading to safety hazards like overheating, fires, or reduced performance. The BMS effectively acts as the brain of the battery pack, coordinating its charge and discharge cycles. This is especially important because electric vehicle batteries typically operate within specific voltage and temperature ranges. When these parameters are not maintained, it could degrade battery health and significantly impact the range and performance of the vehicle.
Key Functions
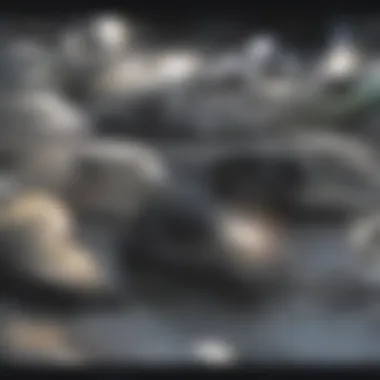
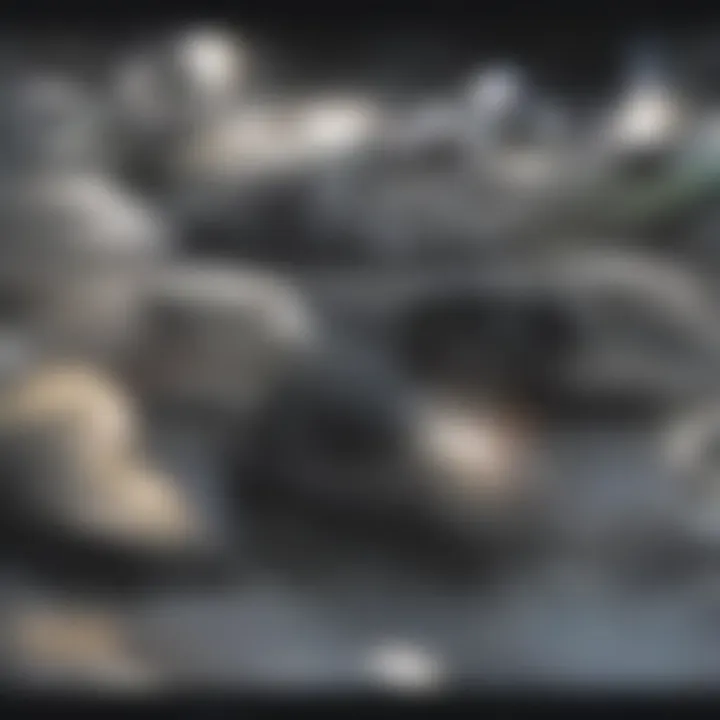
Monitoring
Monitoring is an essential function of a BMS. It involves constantly assessing the voltage, current, and temperature of individual cells within a battery pack. This data is crucial, as it enables the system to prevent conditions that could lead to excessive heat or over-voltage situations. One key characteristic of monitoring is its real-time data acquisition, which helps in making immediate adjustments to the charging and discharging process. This capability is beneficial as it increases the reliability and safety of the battery. A unique feature of monitoring systems includes their capacity for remote diagnostics, allowing for predictive maintenance. This can prevent issues before they manifest as failures.
Balancing
Balancing is another critical element of a BMS. It assures that all cells in a battery pack charge and discharge uniformly. Imbalances can occur due to variations in cell capacity or environmental conditions, risking battery life and performance. The key characteristic of balancing is its ability to equalize the state of charge (SoC) among cells. This function is especially beneficial as it promotes uniform wear on the battery, prolonging its lifespan. A unique feature of balancing systems is their approach to passive and active balancing methods, allowing for adjustments without significant loss of energy, ensuring optimal battery use across all cells in the pack.
Protection
Protection mechanisms in a BMS are vital to safeguarding the battery from potential damage. They involve protections against over-voltage, over-current, and over-temperature scenarios. The key characteristic of protection features is their ability to initiate cut-off signals to prevent dangerous conditions. This makes it a popular choice in electric vehicles since safety is paramount. A unique element of protection systems is their integration with mechanical fuses or circuit breakers to enhance physical safety measures. Therefore, this component not only protects the battery but also serves as peace of mind for users and manufacturers alike.
The Battery Management System is fundamentally essential for electric vehicles, ensuring performance, longevity, and safety of the battery packs.
Charging Infrastructure
Charging infrastructure plays a crucial role in the acceptance and feasibility of electric vehicles. As more consumers consider electric cars, the availability of reliable and accessible charging stations becomes essential. Charging infrastructure encompasses the various types of charging solutions and their locations. It impacts how efficiently users can recharge their vehicles and ultimately influences the practicality of owning an electric vehicle. In this section, we will explore different charging types and the challenges associated with developing extensive charging networks.
Charging Types
Level Charging
Level 1 charging utilizes a standard household outlet, providing a straightforward way to recharge electric vehicles overnight. This is typically a 120-volt connection, making it the most common charging method for home use. The key characteristic of Level 1 charging is its ease of use. Homeowners can plug their vehicle into a standard electrical outlet, requiring no special installation.
However, this method has its limitations. It generally offers a charging speed of about 2 to 5 miles of range per hour, which can result in long charging times if the vehicle is fully depleted. This makes Level 1 charging a beneficial choice primarily for drivers who can charge overnight or have a low daily mileage. Despite its simplicity, the longer recharge times might not suit everyone.
Level Charging
Level 2 charging uses a 240-volt outlet and is more powerful than Level 1. This type is commonly found in both residential and public charging stations and can charge a vehicle much faster, typically offering about 10 to 60 miles of range per hour. The key characteristic of Level 2 charging is its versatility. It can be installed at home or found in commercial locations like shopping centers.
This charging option is popular among electric vehicle owners due to its faster rate of charge. However, it may require professional installation and can have additional costs associated with setup. Its unique feature is the ability to support a higher rate of charging, which is critical for those who need more range quickly. While faster, it must be noted that it does not provide the instantaneous refueling capability associated with traditional gasoline cars.
Fast Charging
DC fast charging, also known as Level 3 charging, represents the pinnacle of charging technology for electric vehicles. This system delivers a high voltage charge directly to the vehicleβs battery, significantly reducing charging time. Depending on the charging station and vehicle compatibility, it can provide 60 to 100 miles of range in just 20 minutes.
The key characteristic of DC fast charging is its rapid pace, making it highly beneficial for long-distance travel. It enables drivers to refuel their electric vehicles quickly and continue their journeys with less downtime. One unique feature of these stations is that they are primarily found along highways and major travel routes, targeting users who need immediate and extensive charging solutions.
However, the installation of DC fast charging stations can be costly and complex. Additionally, not all electric cars are compatible with DC fast chargers, limiting their use based on vehicle specifications.
In summary, charging infrastructure is integral to the success of electric vehicles. Different charging types have their unique advantages and challenges, making it crucial for users to understand which option best suits their needs.
Infrastructure Challenges
While charging infrastructure is critical, various challenges need addressing. First, there is the issue of accessibility. Many areas lack sufficient charging stations, which can instill range anxiety in potential electric vehicle owners. Second, the integration of renewable energy sources into charging stations can drive costs up but offers significant long-term benefits. Lastly, standardizing charging connectors and protocols remains a hurdle for manufacturers and users alike, complicating the charging experience.
As electric vehicle technology and adoption continue to evolve, overcoming these challenges in charging infrastructure will be key to fostering a viable ecosystem for electric vehicles.
Power Electronics
Power electronics are crucial components of electric vehicles, serving as the interface between the electric motor and the battery. These systems manage the conversion of electrical energy, allowing effective distribution for various vehicle functions. The significance of power electronics lies in their ability to control and optimize the performance of electric motors, which enhances the overall efficiency and responsiveness of the vehicle. Understanding these systems can provide insights into how electric vehicles operate at a fundamental level.
Types of Power Electronics
Inverters
Inverters are essential for converting direct current (DC) from batteries into alternating current (AC) for the electric motor. This conversion is vital because most electric motors function on AC. A key characteristic of inverters is their ability to control motor speed and torque by adjusting the frequency and amplitude of the AC signal. Their importance comes from their role in energy efficiency, allowing the vehicle to maximize performance with minimal energy loss.
One unique feature of inverters is their capability to operate in regenerative braking modes. This allows the vehicle to recover energy during braking and feed it back into the battery. The advantages of this feature include improved energy efficiency and increased driving range. However, inverters can introduce some complexities in the control systems and may require careful calibration to ensure performance efficacy.
Converters
Converters, on the other hand, are responsible for managing the flow of energy between different voltages within the vehicle's electrical system. They convert high-voltage power from the battery down to the lower voltage needed for other components, such as lights and infotainment systems. A significant characteristic of converters is their ability to ensure stable power delivery, which is critical for the operation of peripheral systems in an electric vehicle.
Converters are beneficial due to their role in maintaining system integrity and efficiency. They can minimize energy losses during these transformations, which contributes to the optimal operation of the electric vehicle. However, a potential disadvantage lies in their size; high-capacity converters may take up considerable space, which could be a limitation in some vehicle designs.
Role in Efficiency
The role of power electronics in electric vehicles extends far beyond simple energy conversion. They are instrumental in enhancing the overall efficiency ratings of electric cars. By optimizing the energy flow, they contribute to lower energy consumption without compromising performance. The effective management of electricity leads to longer driving ranges and better performance under varying driving conditions.
In summary, power electronics play a vital role in the efficiency of electric vehicles. Their ability to control energy flow directly impacts the vehicleβs performance, making them indispensable in the modern electric vehicle landscape. As technology advances, the design and function of these components continue to evolve, presenting new opportunities for improving electric vehicle technology.
Thermal Management System
The thermal management system is a critical aspect of electric vehicle design and operation. It ensures that all components, especially the battery and electric motor, function within their optimal temperature range. Effective thermal management can lead to improved performance, extended lifespan, and enhanced safety of electric vehicles. A well-designed system can mitigate the risk of overheating, which is crucial in maintaining the efficiency and reliability of the overall vehicle technology.
Importance of Cooling
Cooling is essential in preventing overheating of EV components. Both the battery and motor generate heat during operation. If the temperature rises too high, it can affect performance. It can also lead to catastrophic failures. Thus, cooling systems are vital to the efficiency and safety of electric vehicles. Proper temperature regulation promotes optimal battery life and can enhance vehicle performance during varied driving conditions.
Heat Exchange Methods
Heat exchange methods can be classified into different categories, mainly liquid cooling and air cooling. Each method has its strengths and weaknesses, and their selection often depends on the specific requirements of the vehicle.
Liquid Cooling
Liquid cooling utilizes a fluid, often a mix of water and antifreeze, to absorb heat from critical components. This method is favored for its efficiency and effectiveness. One key characteristic of liquid cooling is its capability to transfer heat away from components faster than air cooling. This can be particularly beneficial during high-performance scenarios, such as rapid acceleration or during long drives.
Unique feature: Liquid cooling systems often include a radiator to dissipate heat, maintaining optimal operating temperatures. The advantages of this system include better thermal regulation and the ability to handle larger heat loads. However, potential disadvantages involve increased complexity and weight of the cooling system.
Air Cooling
Air cooling relies on ambient air flow to dissipate heat from components. This method is generally simpler and lighter than liquid systems. A key characteristic of air cooling is its low maintenance requirement since there are fewer parts that can fail compared to liquid systems.
Unique feature: Air cooling does not require a pump or reservoir, making it a more straightforward option. The advantages of air cooling include lower weight and simplicity. However, its disadvantages are more pronounced in demanding performance situations, where it may not cool as effectively as liquid cooling. Overall, air cooling can be sufficient under normal operating conditions but might not provide the same level of performance under stress.
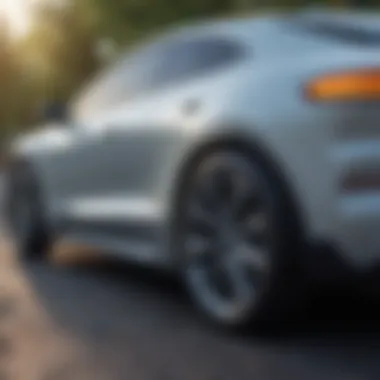
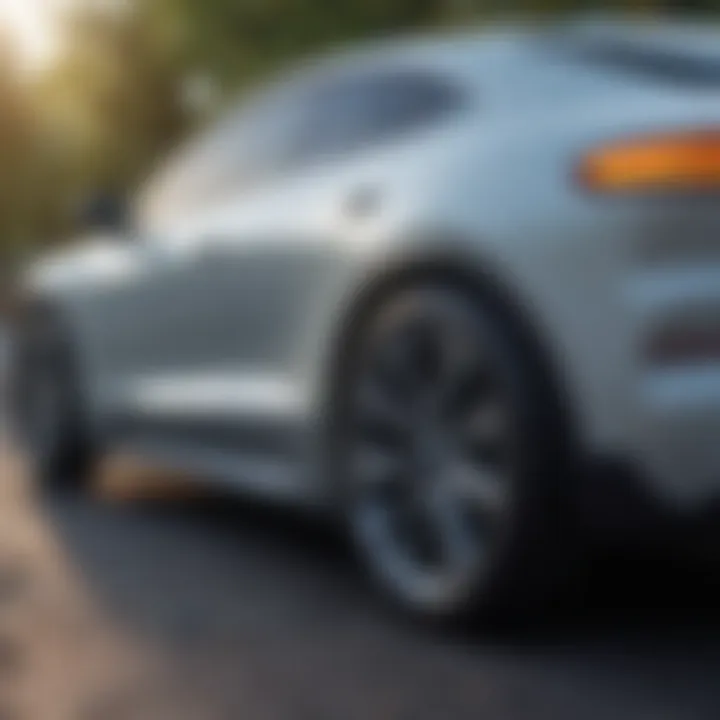
The choice between liquid cooling and air cooling often depends on the desired balance between performance, complexity, and cost in electric vehicle design.
Drivetrain Configuration
The drivetrain configuration in electric vehicles plays a crucial role in both performance and efficiency. It serves as the link between the electric motor and the wheels, determining how power is distributed. Understanding the types of drivetrains and their impacts on vehicle dynamics is essential for anyone interested in electric vehicle technology.
Types of Drivetrains
Single Motor
A single motor configuration uses one electric motor to drive either the front or rear wheels. This setup simplifies the vehicle's design and reduces weight. The main feature of a single motor is its straightforward power delivery, making it a popular choice for many electric vehicles.
One advantage of single motors is the cost savings associated with fewer components required for installation. Vehicles equipped with single motors tend to be lighter, which directly contributes to energy efficiency. However, a potential downside is that a single motor may not provide the same level of performance and traction control compared to dual motor setups.
Dual Motor
In contrast, a dual motor configuration integrates two electric motors, commonly placed on both the front and rear axles. This arrangement enhances traction and stability, especially in adverse driving conditions. The distinctive characteristic of dual motors is their ability to offer all-wheel drive, which helps distribute power effectively.
The unique advantage of dual motors lies in their performance. They allow for quicker acceleration and improved handling characteristics. However, the increased complexity and weight compared to single motor systems can raise costs. Thus, while dual motors deliver superior driving dynamics, they may not be the optimal choice for every situation.
Impact on Handling
Drivetrain configuration significantly affects vehicle handling. In single motor systems, weight distribution is simpler, which can lead to predictable handling during cornering. However, the lack of front-to-rear torque vectoring can lead to understeer in some scenarios. Meanwhile, dual motor setups provide enhanced stability and cornering performance.
Both configurations present unique advantages and challenges based on the designed use and intended audience of electric vehicles. Careful consideration of each drivetrain type is vital when designing electric vehicles, as it impacts overall driving experience and consumer satisfaction.
Chassis and Body Design
The chassis and body design of electric vehicles play a crucial role in their overall performance, safety, and efficiency. This component serves as the structural framework that supports the electric vehicleβs weight and houses various essential parts like the battery and motor. A well-designed chassis can enhance stability and handling, contributing to a more enjoyable driving experience. Moreover, the body design impacts aerodynamics, which can significantly influence the vehicleβs range.
Material Considerations
The choice of materials used in chassis and body construction is fundamental for several reasons. First, lightweight materials, such as aluminum or carbon fiber, reduce the overall weight of the electric vehicle. This reduction directly correlates with improved energy efficiency, as less energy is required to accelerate a lighter vehicle.
Changes in the automotive industry have led to the adoption of advanced materials. For example, high-strength steels are utilized for their durability and cost-effectiveness. By combining different materials, manufacturers can achieve a balance between weight, strength, and safety. When evaluating the materials, manufacturers must also consider production costs and environmental impacts. This leads to choices that support sustainability while ensuring consumer safety.
Aerodynamics in EV Design
Aerodynamics is another vital aspect of chassis and body design that deserves attention. The aerodynamic efficiency of a vehicle affects how it cuts through the air, which in turn influences energy consumption. An optimized shape can reduce drag coefficients, allowing the vehicle to travel farther on a single charge.
Improving aerodynamics involves various strategies. Smooth body contours, reduced frontal area, and strategically placed spoilers can all contribute to better airflow around the vehicle. Electric vehicle designers often engage in wind tunnel testing to refine these shapes. Additionally, elements like active grille shutters can adjust based on speed, further enhancing aerodynamics.
"The importance of a well-thought-out chassis and body design cannot be understated. It represents a synthesis of engineering disciplines that ensures safety, efficiency, and performance."
Control Systems
Control systems are critical in the functionality and efficiency of electric vehicles. They regulate various functions, including motor control, energy consumption, and interaction with user interface systems. Their role is crucial, as they help optimize the performance of electric vehicles, ensuring smooth operation under different conditions.
Types of Control Algorithms
Control algorithms are mathematical models and strategies that dictate how systems respond to inputs. In electric vehicles, these algorithms play a significant role in managing the behavior of electric motors and other components. Various types include:
- PID Control: Proportional-Integral-Derivative control helps in fine-tuning the motor speed and torque by adjusting output based on current error, past error, and predicted future error.
- Fuzzy Logic Control: This type mimics human decision making by handling approximate reasoning. It manages variables that are not easily quantified, making it suitable for dynamic driving conditions.
- Model Predictive Control (MPC): MPC uses a model of the vehicle to predict future behavior and apply control inputs to optimize performance, which is critical in high-speed driving or during rapid acceleration.
The choice of algorithm impacts efficiency and safety by determining responsiveness and handling characteristics of the vehicle.
Integration with Other Components
The effectiveness of control systems relies heavily on how well they integrate with other components of the electric vehicle. For instance, a control system must work cohesively with the power electronics that convert and control electrical energy flow. Additionally, they interface with the battery management system to monitor battery status and optimize charging cycles. Such integrations must consider:
- Real-time Data Processing: Control systems need to process information from sensors quickly. This allows immediate responses to changes in driving conditions.
- Communication Protocols: Different components often use various protocols. Standardizing these ensures smooth data exchange. A common standard is CAN bus, which reduces the risk of communication issues.
- Safety Features: Integration is also necessary for implementing safety measures effectively. Control systems can activate safety protocols during critical failures or accident scenarios, thus protecting the vehicleβs occupants.
The role of control systems extends beyond mere operational efficiency. They are central to the safety, reliability, and overall experience of electric vehicle ownership. As technology advances, these systems will evolve further, integrating more sophisticated algorithms to handle increasingly complex driving scenarios.
The integration of advanced control systems in electric vehicles ensures they operate safely and efficiently while meeting the demands of modern transportation.
Regenerative Braking System
The regenerative braking system is a significant component in electric vehicles, improving both performance and efficiency. This technology converts kinetic energy, produced during deceleration, back into stored energy in the vehicleβs battery. Unlike traditional braking systems that merely dissipate energy as heat, regenerative braking utilizes this kinetic energy, leading to better battery range and reduced wear on mechanical braking components.
Mechanics of Regenerative Braking
Understanding the mechanics behind regenerative braking involves recognizing its operational principles. When a driver applies the brakes, the electric motor runs in reverse. This process slows down the vehicle while simultaneously generating electricity. The energy generated is directed back to the battery, replenishing its charge.
Key components involved include:
- Electric Motor: Functions both to drive the vehicle and act as a generator.
- Power Electronics: Control the flow of electricity between the motor and battery, ensuring efficient energy transfer.
- Battery Management System: Monitors and manages the energy retrieved, ensuring optimal storage capacity.
The efficiency of this system means that on average, an electric vehicle can recover about 10-70% of the energy that would otherwise be lost. Many electric vehicles allow drivers to feel a degree of deceleration without using the brake pedal, enhancing control during driving.
Benefits for Efficiency
Regenerative braking offers several benefits that contribute to the overall efficacy of electric vehicles. Among these benefits are:
- Increased Driving Range: By converting a portion of kinetic energy back into usable energy, regenerative braking extends the distance that an electric vehicle can travel on a single charge.
- Reduced Energy Consumption: The system reduces the reliance on stored energy. This result lowers energy costs and promotes longer battery life.
- Enhanced Vehicle Control: Regenerative braking provides smoother deceleration, improving the comfort and safety for passengers.
- Resource and Cost Efficiency: It decreases maintenance costs associated with conventional brake systems since it reduces wear.
"Regenerative braking is a critical technology that defines the difference between conventional and electric vehicular systems."
For further reading on the technologies related to electric vehicles, you may refer to resources like Wikipedia, Britannica, and discussions on Reddit about electric vehicle innovations.
User Interface Systems
User Interface Systems play a key role in the overall experience of driving electric vehicles. These systems serve as the bridge between the driver and the carβs functionalities, allowing for efficient interaction with multiple features. Their importance cannot be overstated because they enhance the usability and accessibility of vehicles, making advanced electric technology more approachable for all users.
Dashboard Technology
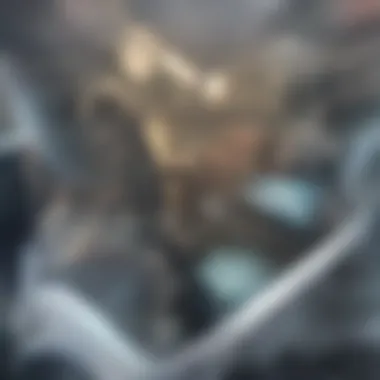
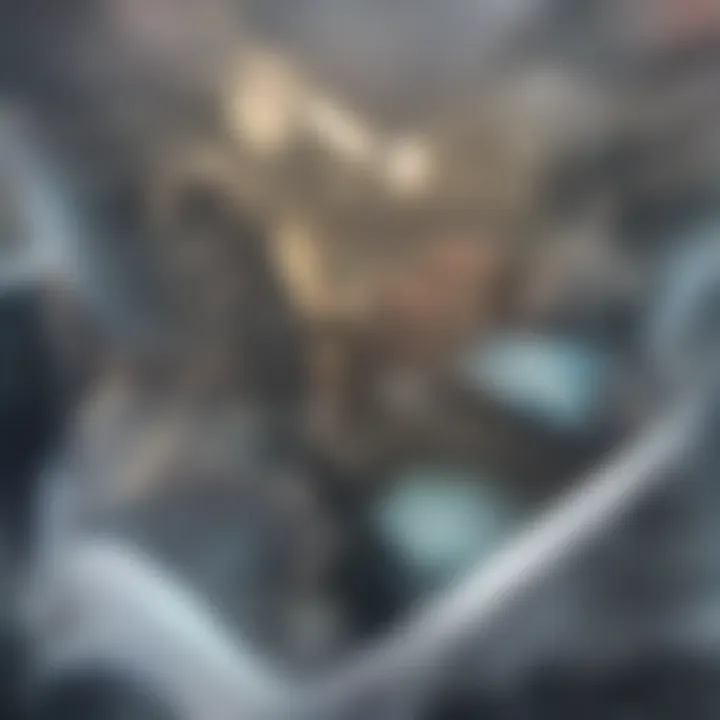
The dashboard is perhaps the most critical part of the User Interface Systems. This is where drivers receive important information about the vehicleβs performance and status. Modern dashboards in electric vehicles are increasingly digital, featuring high-resolution displays that offer various metrics.
Typical elements found on a dashboard include:
- Speedometer and battery charge indicator: These help the driver track how fast they are going and how much charge remains.
- Navigation systems: Integrated maps and directions make it simple to find the best routes.
- Real-time diagnostics: Information about the vehicle's health can alert drivers to maintenance needs or any operational issues.
Users benefit from a clean layout, which aids in minimizing distractions while driving. Customization of the dashboard also allows drivers to prioritize what information is most relevant to them, improving the overall experience.
Connectivity Features
Connectivity features form an integral part of User Interface Systems by enabling seamless communication between the vehicle, the driver, and the external environment. Most electric cars today come with various connectivity options. These include:
- Bluetooth: Allows hands-free calling and music streaming.
- Wi-Fi: Provides internet access in the vehicle, allowing for software updates and other online features.
- Smartphone integration: Systems like Apple CarPlay and Android Auto allow drivers to mirror their phoneβs features on the car's dashboard display.
The benefits of these connectivity features are substantial. They provide:
- Enhanced Safety: Keeping hands on the wheel and eyes on the road while accessing essential functions.
- Increased Convenience: Anywhere and anytime access to maps, music, and other applications.
- Remote Vehicle Management: Some systems allow users to check battery status, lock or unlock the car, or even pre-condition the interior temperature before entering.
Connecting the dashboard technology with these features results in a more cohesive user experience, enabling drivers to maximize their electric vehicle's potential. In essence, well-designed User Interface Systems are essential to making electric vehicles practical and enjoyable for everyday use.
"A well-designed user interface is critical for the success of electric vehicles, as it enables drivers to fully utilize advanced technologies without becoming overwhelmed."
Safety Components
Safety components are crucial in electric vehicles (EVs), as they play a significant role in ensuring the well-being of both passengers and pedestrians. Given that EVs operate differently than conventional gasoline-powered vehicles, incorporating advanced safety technologies is imperative. The safety components not only focus on crash avoidance but also on minimizing injuries and damage during accidents. These elements provide reassurance to consumers, which is essential for wider adoption of electric vehicles.
Crash Safety Technologies
Crash safety technologies are designed to protect vehicle occupants in the event of a collision. This includes a combination of structural integrity, advanced materials, and safety systems.
- Structural Integrity: The chassis and framework of electric cars are engineered to absorb impact energy. This design reduces the force transmitted to passengers during a crash.
- Crumple Zones: These areas are strategically designed to deform during an accident, further absorbing energy and minimizing injury risks.
- Airbags: Advanced airbag systems consider the lower weight of electric cars. Innovative deployments and more sensitive sensors contribute to effective performance in different crash scenarios.
Recent advancements include integration of sensors and algorithms that monitor various factors, like speed and impact angles, enabling the vehicle to provide real-time feedback. Such technology can trigger preemptive actions to mitigate collision outcomes.
"The integration of crash safety technologies is vital for consumer confidence and safety assurance."
Fire Safety Measures
Electric vehicles, like all vehicles, are susceptible to fire risks. However, the battery systems in EVs introduce unique challenges and considerations.
- Thermal Management: Proficient thermal management systems are critical. They regulate battery temperature to prevent overheating, which can lead to thermal runaway and fires.
- Battery Enclosures: Robust and heat-resistant enclosures protect battery cells, minimizing fire risk during accidents or thermal events.
- Fire Suppression Systems: Certain electric car models integrate fire suppression technologies in the battery pack, which helps to extinguish any potential fires quickly.
Training for first responders is also essential so that they can efficiently manage electric vehicle fires. Understanding the differences in risks associated with battery-powered vehicles facilitates better emergency responses.
Sustainability in Electric Vehicle Production
Sustainability in electric vehicle (EV) production is a critical aspect that reflects the broader goals of reducing environmental impact and advancing sustainable technology. As the demand for electric cars grows, it is vital to consider how these vehicles are made, focusing on their entire lifecycle from material sourcing to end-of-life disposal. With the rising awareness of climate change and resource depletion, the automotive industry faces pressure to adopt practices that prioritize sustainability.
Material Sourcing
Material sourcing for electric vehicles involves selecting materials that are both environmentally friendly and efficient in terms of performance. Electric cars rely on various components, such as batteries, electric motors, and chassis materials. Each of these components requires specific materials that pose challenges in their extraction and processing.
Factors such as the origin of raw materials, the impact of mining, and the production processes must be evaluated. For instance, lithium-ion batteries, commonly used in EVs, require lithium, cobalt, and nickel. The extraction of these materials can lead to significant environmental degradation and social issues in mining regions. Striking a balance between ensuring an adequate supply of materials and minimizing environmental damage is essential.
Being responsible in material sourcing also involves using recycled materials whenever possible. A circular economy approach can significantly reduce the ecological footprint of EV production. For example, using recycled aluminum for vehicle bodies not only decreases the demand for new bauxite but also cuts energy consumption in the manufacturing process. Furthermore, the automotive industry is exploring more sustainable alternatives, like bio-based materials, that can replace traditional materials without compromising safety and performance.
End-of-Life Considerations
End-of-life considerations are an important part of the sustainability equation in electric vehicle production. Considering what happens to a vehicle after its useful life offers insight into the complete lifecycle management of the car. Proper disposal and recycling can significantly mitigate the environmental impact of electric vehicles.
EV batteries, in particular, present challenges at end-of-life. As they age, their capacity diminishes, raising the need for safe disposal methods and recycling programs. Proper recycling processes can recover critical materials, which can then be reused to create new batteries or other components. This not only conserves natural resources but also reduces the need for further mining activities and minimizes toxic waste.
Moreover, manufacturers are beginning to design vehicles with disassembly in mind. This facilitates the recycling process and increases the efficiency of reclaiming valuable materials. Design choices about modular construction allow for easier upgrades and replacements, extending the vehicle's overall lifecycle.
"To achieve true sustainability, it is essential to consider each stage of an electric vehicleβs lifecycle, from production through to recycling."
Ensuring a sustainable production process contributes to the broader objectives of reducing carbon emissions and promoting a more circular economy in the automotive sector. As electric vehicle technology continues to evolve, it is crucial for industry stakeholders to prioritize sustainability to meet consumer expectations and regulatory requirements, ultimately leading to a greener future.
Future Trends in Electric Vehicle Technology
The field of electric vehicle technology is continuously advancing, shaped by evolving consumer expectations and regulatory frameworks. Understanding these trends is critical, as they will directly influence the design, development, and adoption of electric vehicles (EVs). Technology advancement is vital for improving performance, enhancing user experience, and reducing environmental impact. Contributing to this landscape of change are several emerging technologies and anticipated market shifts that hold significant implications for the future of transportation.
Emerging Technologies
Numerous new technologies are emerging within the realm of electric vehicles. Here are some notable ones:
- Solid-state Batteries: Unlike conventional lithium-ion cells, solid-state batteries promise better energy density and safety. Their solid-electrolyte structure reduces risks of fire and prolongs lifecycle.
- Vehicle-to-Grid (V2G) Systems: This system allows EVs to not just consume energy but also feedback power into the grid. It can stabilize the grid during peak demand and provides financial benefits to users.
- Smart Charging Solutions: These solutions enable vehicles to charge during off-peak hours or when renewable energy sources produce excess power. Smart charging systems optimize energy use, thus minimizing costs and impact.
- Advanced Driver-Assistance Systems (ADAS): As EVs often come with notable tech features, the integration of ADAS for better safety and convenience continues to evolve. These systems will enhance user experience and drive further acceptance of electric vehicles.
Incorporating these innovations into everyday electric cars aligns with the growing demand for cleaner, smarter, and more efficient transportation options.
Predicted Market Developments
The electric vehicle market will undergo substantial transformation in the coming years. Analysts predict several key developments:
- Increase in EV Adoption: The shift towards electric vehicles will accelerate as more consumers become eco-conscious. Incentives from governments are likely to enhance this trend.
- Expansion of Charging Infrastructure: As the number of EVs increases, expanding charging infrastructure will be crucial. Investments in fast-charging stations will offer consumers convenience and confidence in their buying decisions.
- Diversity in EV Models: Consumers will increasingly find a wider variety of vehicle models, including trucks and SUVs, that cater to diverse preferences. Manufacturers will feel the pressure to provide options that suit different lifestyles.
- Enhanced Recycling Strategies: As the demand for batteries grows, so too will the conversation around sustainable end-of-life recycling solutions. Companies will likely focus on battery reuse to mitigate waste.
"The future competitiveness of the automotive industry centers on innovation in electric vehicle technology and infrastructure."
In summary, the trends in electric vehicle technology indicate a proactive movement toward sustainable and advanced solutions. From pioneering technologies like solid-state batteries to market predictions on adoption and infrastructure, each aspect builds towards a cohesive future in transportation. Understanding these components not only enhances awareness but also informs strategic decisions for stakeholders in the industry.
End
Exploring the components of electric cars is not merely an academic exercise; it holds practical significance for various stakeholders. As the transition towards electric mobility becomes increasingly prevalent, understanding these components aids in comprehending the technological advancements driving this shift. Every aspect, from the electric motor to the battery management system, plays a vital role in ensuring efficiency, sustainability, and performance of electric vehicles.
Summary of Key Components
In this article, we have delved into several crucial components that constitute electric vehicles. The electric motor serves as the driving force, converting electrical energy into mechanical energy. The battery technologies, predominantly lithium-ion and emerging solid-state batteries, are essential for energy storage and optimizing driving range. We also examined the battery management system, which guarantees the safety and longevity of the vehicle's most vital component. Each of these elements interplays with power electronics, thermal management, and control systems, creating a complex yet efficient ecosystem.
Implications for Future Research
The findings presented expose several avenues for future research. As technologies evolve, continuous improvement in battery performance can lead to enhancements in driving range and efficiency. Research into sustainable production practices can also help mitigate the environmental impact of electric cars. Furthermore, exploring the integration of artificial intelligence in control systems may unlock new potentials in vehicle automation and user experience. Future studies can deepen our understanding of these components' roles, refining existing technologies and facilitating innovative solutions in the electric vehicle landscape.
"As technology progresses, the synergy between components within electric vehicles will become increasingly pivotal."