Understanding CRISPR: Mechanisms and Applications
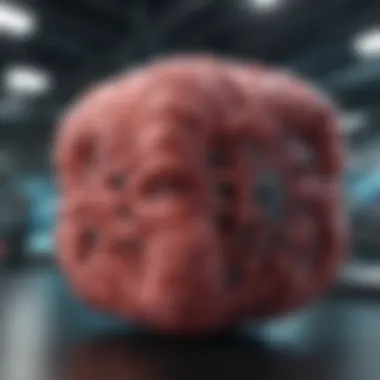
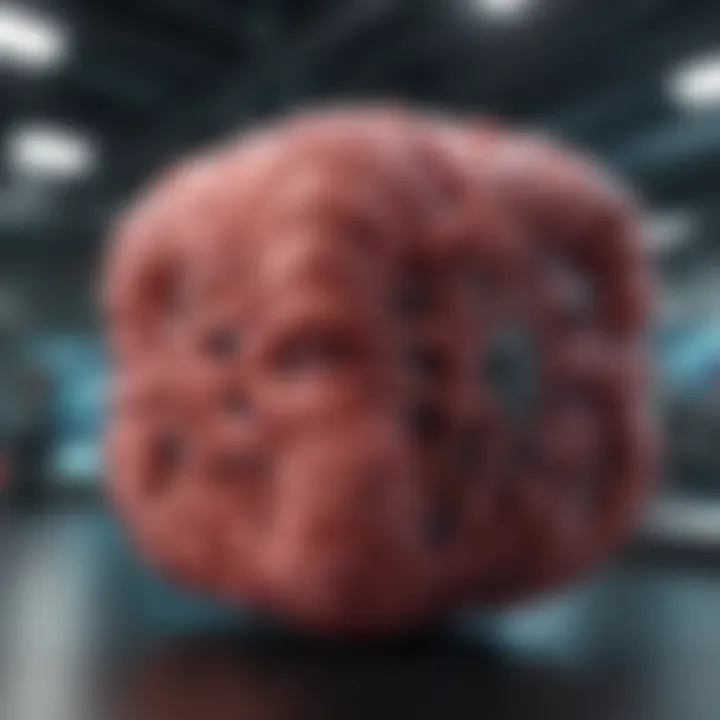
Intro
The remarkable development of CRISPR technology has taken the scientific world by storm, revolutionizing how we perceive genetic manipulation. At its core, CRISPR offers an efficient, precise, and relatively simple means of altering DNA sequences, leading to advancements in fields such as medicine, agriculture, and biological research. The simplicity of its mechanism belies its profound implications, ranging from the potential eradication of genetic diseases to enhancing crop resilience.
Understanding how CRISPR operates is not merely an academic pursuit—it's a vital step toward harnessing its full potential. This exploration aims to dissect the intricacies of these gene-editing tools, laying bare the mechanisms that make CRISPR so powerful. What constitutes these components? How do they interact? This thorough examination serves as a roadmap through the complex landscape of gene editing, shedding light on a technology that could reshape our biological future.
Research Context
Background Information
CRISPR, which stands for Clustered Regularly Interspaced Short Palindromic Repeats, initially emerged from the immune system of bacteria, which use it to fend off viral attacks. In essence, CRISPR incorporates snippets of viral DNA into its own genetic material, creating a sort of ‘memory’ of their past invaders. When re-introduced back into these bacteria, CRISPR is capable of recognizing and cutting the viral DNA if it attempts to invade again. This natural defense mechanism laid the groundwork for its adaptation as a gene-editing tool, sparking a wave of innovation and research since its clear demonstration of applicability in eukaryotic cells in 2012.
Importance of the Study
Delving into CRISPR technology is crucial for several reasons. Firstly, it represents a significant leap in our capability to edit genomes with unprecedented precision. This has immediate applications in treating genetic disorders like cystic fibrosis, sickle cell disease, and even certain forms of cancer. Secondly, the agricultural sector stands to benefit enormously; CRISPR can create crops that withstand harsh environmental stresses, thus improving food security. Moreover, understanding CRISPR's mechanics can illuminate ethical implications, guiding informed discussions about genetic modification and its societal impact. Drawing connections between the underlying science and its applications can bridge gaps between research, policy-making, and public discourse.
Discussion
Interpretation of Results
Given the myriad applications of CRISPR, interpretations of its results vary widely. In clinical contexts, the ability to target and modify genes presents a profound shift in disease treatment. Case studies illustrating successful edits have shown reduction in symptoms or even complete remission in some genetic disorders. Meanwhile, in agriculture, successful CRISPR interventions have yielded crops that are not just heartier, but also more nutritious. These successes showcase the technique's practical benefits and invite further exploration of its limitations.
Comparison with Previous Research
Innovations in gene editing pre-CRISPR involved tools like ZFNs (Zinc Finger Nucleases) and TALENs (Transcription Activator-Like Effector Nucleases). While effective, they were comparatively cumbersome, requiring extensive design and testing phases. CRISPR, on the other hand, simplifies the process significantly, making gene editing more accessible. Additionally, the cost associated with CRISPR technology is typically lower, which allows a wider range of researchers and institutions to explore its potentials.
Understanding CRISPR: An Overview
CRISPR has revolutionized the field of biotechnology, driving research and applications in ways that were the stuff of science fiction a couple of decades ago. It has become increasingly important for educators, students, scientists, and professionals to grasp this chapter of modern biological science. Delving into CRISPR equips individuals with knowledge about how gene editing can affect everything from curing diseases to enhancing agricultural resilience. This overview sets the stage for a deeper dive into the mechanisms of CRISPR.
Historical Context
The journey to understanding CRISPR technology began in the 1980s, when researchers first noted peculiar repetitive sequences in bacterial DNA. The breakthrough came with the work of scientists like Francisco Mojica, who recognized these sequences as a sort of immune memory for bacteria against viruses. Fast forward to 2012; that’s when Jennifer Doudna and Emmanuelle Charpentier transformed these observations into a practical gene-editing tool. This leap from discovery to application illustrates how science often builds on prior knowledge. Today, CRISPR stands at the forefront of molecular biology, reshaping our understanding of genetics.
What is CRISPR?
The term CRISPR stands for "Clustered Regularly Interspaced Short Palindromic Repeats." It’s a mouthful, indeed. But its essence is quite simple.
Definition of CRISPR
CRISPR refers to a unique system used by bacteria to fend off viral infections, and when adapted for human use, it monitors DNA sequences precisely. In this context, it opens a floodgate of possibilities in gene editing, allowing scientists to edit specific DNA sequences with high accuracy. One of its most significant characteristics is its adaptability. Researchers can design CRISPR systems tailored for myriad applications—this versatility makes it a massively favorable tool in modern science.
The unique feature of CRISPR is its capability to target specific genetic sequences without affecting adjacent genes, effectively reducing the unpredictability often associated with other gene-editing methods. This specificity is a game-changer for therapeutic applications where off-target effects can be detrimental.
Components of CRISPR Technology
CRISPR doesn’t work in isolation; it comprises several components essential for its function. Understanding these is key to grasping how CRISPR operates effectively.
The primary components are the Cas9 enzyme and guide RNA. The Cas9 enzyme functions like molecular scissors, cutting DNA at the designated location. Meanwhile, the guide RNA is responsible for finding the right target within the DNA strand. This separation of functions allows for efficient editing of genetic material.
The elegant simplicity of these components comes with substantial advantages. The combined efficacy of Cas9 and guide RNA ensures not only precision but also a streamlined process, allowing for faster and more reliable gene editing. That said, challenges remain, including optimizing the guide RNA design for various target sequences and potential immune responses to the components themselves.
Importance of CRISPR in Modern Science
CRISPR has become a cornerstone of modern genetic research and application. Merely looking at its potential in medicine is eye-opening. Scientists can use it to target genetic disorders, develop more effective cancer treatments, and even explore the possibilities of gene therapy. In agriculture, CRISPR enables the creation of crops resistant to pests and environmental stresses, thereby ensuring food security.
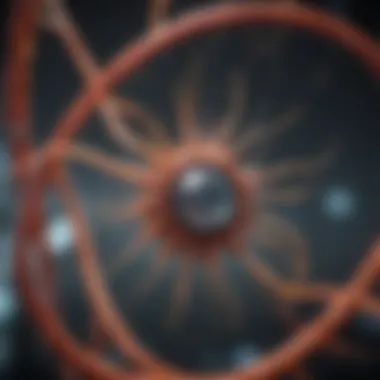
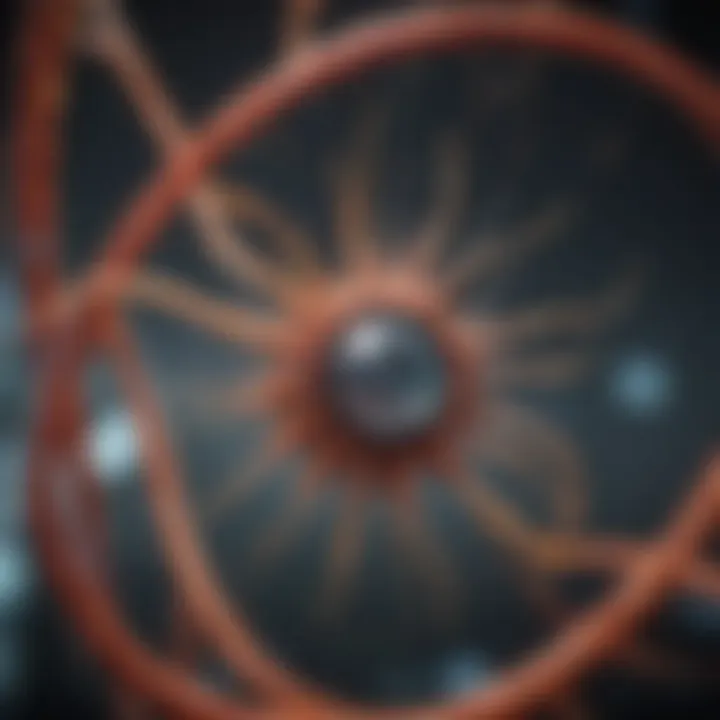
The impact of CRISPR transcends individual disciplines, influencing ethics, policy-making, and public perception. As these technologies empower us to manipulate life at a fundamental level, they also prompt important discussions about moral implications and safety. Balancing the benefits with ethical considerations shapes CRISPR’s future trajectory in science and society.
In summary, this overview not only sets the groundwork for understanding CRISPR but also highlights its vast realms of implications, affirming its role as an indispensable technology in contemporary research. By examining the intricate mechanisms of CRISPR, we can appreciate its breadth and nuance, framing future inquiries and applications on solid foundations.
How CRISPR Functions
Understanding how CRISPR functions is crucial for grasping its transformative effect across numerous fields. This section delves into the intricate mechanics behind CRISPR technology, elucidating how it achieves gene editing with a precision that was once thought impossible. By exploring the core components and the specific processes involved in gene editing, readers can better appreciate the potential benefits and considerations that arise as this technology continues to develop.
The Core Components
In any advanced technology, the foundational elements are paramount to its success. In CRISPR, two key components stand out: the Cas9 enzyme and the guide RNA. Each of these plays a distinctive role, forming an indelible partnership necessary for effective gene editing.
Cas9 Enzyme
The Cas9 enzyme acts as the cutting scissors in the CRISPR system, playing a vital role in the actual gene editing process. This enzyme's ability to recognize and cut DNA at specific sites is what sets it apart. A notable characteristic of Cas9 is its dual-targeting flexibility, allowing it to attach to almost any DNA sequence that matches its design. This versatility makes it a crowd favorite among scientists working with gene editing.
However, it does have downsides. For example, while Cas9 is efficient, off-target effects can occur, potentially leading to unintended modifications in the genome. Despite this, its overall utility and the benefits it provides far outweigh these concerns. Cas9's efficiency makes it a leading choice for researchers eager to push the boundaries of genetic manipulation.
Guide RNA
Guide RNA is like the GPS of the CRISPR system, directing Cas9 to the specific location on the DNA strand. This RNA component is designed to complement the target DNA sequence, ensuring accuracy during the editing process. One of the key traits of guide RNA is its capacity to be engineered to target virtually any gene, which expands the horizons of potential modifications.
On the flip side, if the guide RNA is not properly designed, it can misdirect the Cas9, resulting in off-target cuts. Hence, it’s crucial that careful thought goes into constructing the guide RNA, emphasizing the balance of precision with innovation. Ultimately, guide RNA is indispensable within the CRISPR framework, providing the targeted approach for the entire system.
The Gene Editing Process
The gene editing journey undertaken by CRISPR involves several meticulously orchestrated steps, each playing its part in ensuring the overall effectiveness of the process.
Step One: Designing the Guide RNA
Creating a successful guide RNA is crucial to the efficacy of the CRISPR system. This initial step involves selecting the desired target sequence and crafting an RNA strand that complements it. The efficiency of this step holds great weight, as a well-designed guide RNA translates to improved targeting accuracy. Its adaptability means that this design process can flow smoothly into various applications, from targeting diseases to enhancing crop traits.
Nevertheless, it also comes with its challenges. Crafting a precise guide RNA requires not only technical skill but also an understanding of the target DNA's surrounding environment. The risk of off-target effects looms here as well, making careful planning essential. The advantages brought by a robust guide RNA strategy are significant, yielding the potential for a more effective gene-editing process.
Step Two: Introducing the CRISPR System into Target Cells
Once the guide RNA is designed, the next phase is introducing the entire CRISPR system into the target cells. Methods such as electroporation or lipid-based delivery systems can be utilized, with each having its pros and cons. This step plays a significant role, as the efficiency of cell uptake can affect the overall outcome.
A clear advantage of this stage is the potential to modify cells without altering the host organism in drastic ways. However, any delivery method comes with its set of complications, including cost and potential cellular damage. That's why researchers continue to pioneer better delivery systems to decrease side effects.
Step Three: Cas9 Binding and Cutting
By this third step, the Cas9 enzyme binds to the guide RNA and locates the target DNA sequence, ready to make its incision. This binding process is pivotal; it represents the marriage of two components that together work to achieve gene editing. The Cas9 precisely cuts at the desired location, initiating the modification process.
The beauty of this step lies in the speed and accuracy with which Cas9 operates. That said, if there are mismatches or errors, this could result in unwanted cuts. Until the precision can be assured, monitoring success rates is critical for future improvements.
Step Four: DNA Repair Mechanisms
After the DNA is cut, the cell plays its hand in the final act of gene editing. The natural repair mechanisms of the cell work to mend the break, which can lead to various outcomes. Researchers can take advantage of this by directing it to either introduce specific mutations or to correct existing errors.
The advantageous side of the DNA repair stage is its inherent capability to harness the cell’s natural functions for beneficial outcomes. However, the non-specific nature of these repair mechanisms can lead to unpredictable results, as not every repair process occurs as planned. Thus, it is essential to optimize conditions to guide the desired outcomes more effectively.
In summary, understanding how CRISPR functions lays the groundwork for its effective use in gene editing. Comprehending the roles of Cas9, guide RNA, and the editing process reveals how far we’ve come and where the technology might lead.
Applications of CRISPR Technology
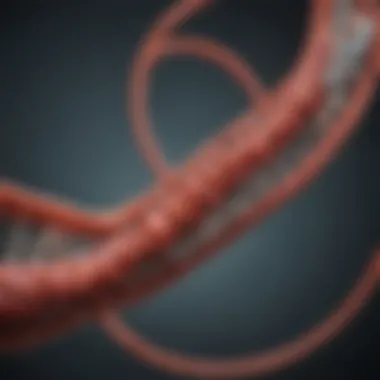
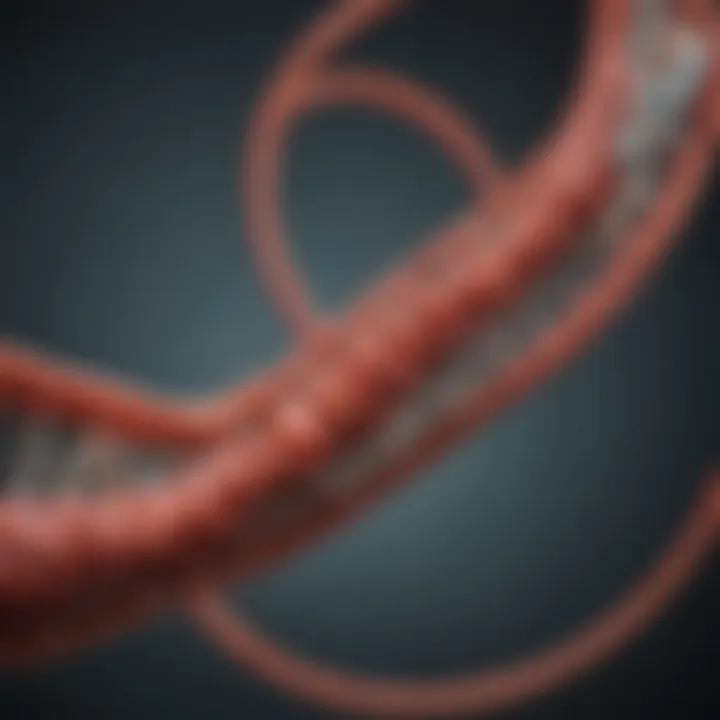
CRISPR technology has become a cornerstone in various fields, significantly altering how we approach challenges in medicine, agriculture, and environmental conservation. The ability to edit genes with precision opens up a world of possibilities, making it essential for students, researchers, and professionals alike to grasp its vast implications.
Biomedical Applications
Gene Therapy
Gene therapy is a remarkable application of CRISPR that aims to treat or prevent diseases by altering genes within an individual's cells. This method stands out due to its potential to correct mutations that cause genetic disorders. The main characteristic of gene therapy is its tailored approach to address specific genetic conditions, making it a popular choice in modern medicine.
It is beneficial because it offers hope for previously untreatable conditions, such as cystic fibrosis and Duchenne muscular dystrophy.
One unique feature of gene therapy using CRISPR is its ability to provide a one-time treatment that can lead to long-lasting effects, eliminating the need for continuous medication. However, despite its advantages, potential risks exist, such as immune reactions or unintended genetic alterations.
Cancer Treatment
Cancer treatment through CRISPR represents another frontier in biotechnology. The use of this technology allows researchers to target and modify genes responsible for cancer progression. A key characteristic of this approach is its focus on precision, enabling the destruction of cancerous cells while sparing healthy ones. This specificity makes it a promising and beneficial direction in oncology.
Notably, CRISPR can help create personalized therapies tailored to an individual's unique tumor profile, facilitating the development of treatments that could yield better outcomes than traditional approaches. However, challenges remain, including ethical considerations and the need for extensive clinical trials to ensure safety and efficacy.
Agricultural Innovations
Crops Improvement
In agriculture, CRISPR is making waves by enhancing crop resilience and yield. The specific aspect of crops improvement focuses on developing varieties that can withstand harsh conditions such as drought, pests, and diseases. This is vital for food security in changing climate scenarios. A primary characteristic is the speed at which it allows scientists to develop new crop varieties compared to traditional breeding techniques, which can take years.
This technology’s unique feature lies in its precision; CRISPR-based modifications can be made without introducing foreign DNA, keeping the natural integrity of the plant. Although promising, there are regulatory hurdles and public concerns over genetically modified organisms that need addressing.
Animal Breeding
Another exciting aspect of CRISPR in agriculture is its application in animal breeding. This method aims to enhance desirable traits such as growth rates, disease resistance, and reproductive efficiency. The key characteristic of CRISPR in this context is its ability to make precise genetic changes, which significantly speeds up the breeding process.
Creating genetically edited animals can provide advantages in producing livestock that contribute positively to both economic viability and animal welfare. However, ethical ramifications regarding animal welfare and biodiversity must be closely examined as this technology develops.
Environmental Applications
Biodiversity Conservation
Biodiversity conservation is a critical application of CRISPR technology, as it can help manage and protect endangered species. A significant aspect here is the potential to edit genes in species to make them more resilient to environmental changes or diseases. This characteristic of adaptability is essential in a world facing rapid climate change.
The unique feature of this application is the potential to revive extinct species through de-extinction techniques. This could restore ecological balance and contribute to preserving ecosystems. Still, it raises complex ethical and ecological questions about the repercussions of reintroducing species into their former habitats.
Bioremediation
Bioremediation involves using biological agents, like microorganisms, to clean up contaminated environments, and CRISPR can enhance this process. By editing specific genes, scientists can produce microorganisms capable of breaking down pollutants more effectively. A key characteristic of bioremediation in this context is its cost-effectiveness and environmentally-friendly approach compared to conventional methods.
One unique feature is that it can be tailored to target specific contaminants. However, a significant downside is the risk involved if engineered organisms were to spread uncontrollably in the environment, leading to unforeseen ecological impacts.
"The applications of CRISPR technology underscore the potential for transformative advancements across many fields, yet they also remind us to tread carefully in this uncharted territory."
Ethical Considerations Surrounding CRISPR
The advent of CRISPR technology has stirred a pot of complex ethical questions that deserve careful scrutiny. As we delve deeper into gene editing, it becomes vital to assess how this powerful technology will impact not just our immediate scientific landscape but also our societal fabric. Ethical considerations are paramount because they help to shape public perception, assure safety, and guide the responsible development and application of CRISPR.
Potential Risks to Human Health
One of the main concerns regarding CRISPR technology is the potential risks it poses to human health. Gene editing offers remarkable possibilities, but it also raises several alarms about unintended consequences. For instance, the precision of CRISPR is often touted as a major benefit; however, off-target effects, where the system edits unintended parts of the genome, can lead to unforeseen health issues. This unpredictability might manifest in the form of new genetic disorders or other adverse effects, complicating treatment protocols.
Furthermore, there's the worry that edited genes could be passed down to future generations, a scenario dubbed germline editing. If errors occur in these alterations, not only might we face immediate health crises, but the repercussions could echo through generations, leading to a host of inherited conditions. These risks are not merely speculative. They stress the necessity for rigorous testing and monitoring as gene editing techniques advance and expand into human therapeutics.
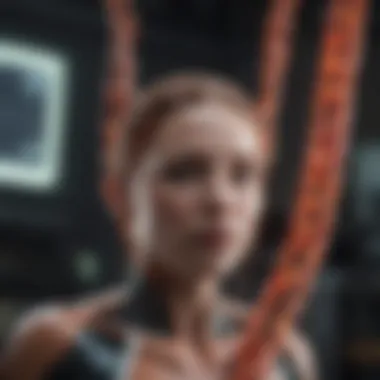
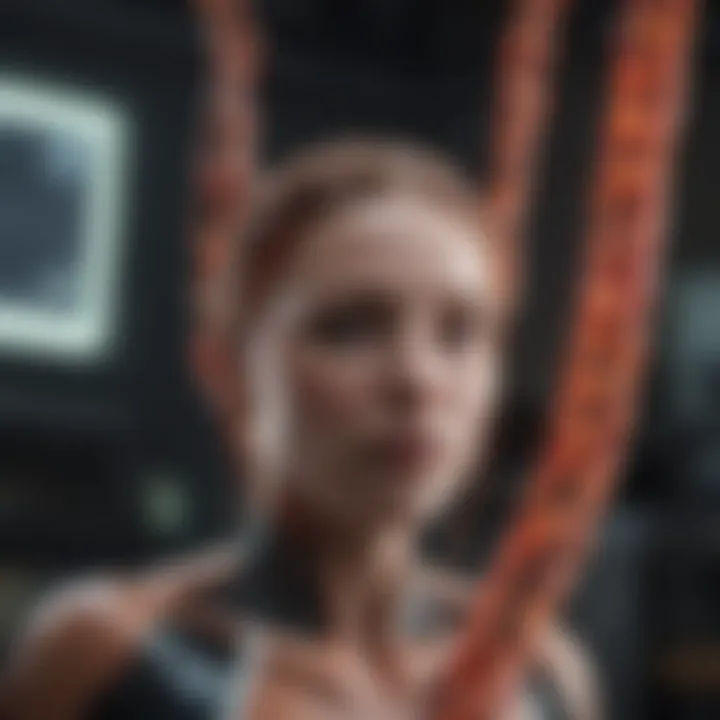
"As we tread into the unknown waters of genetic modification, we must ask ourselves: what do we do if we awaken a Pandora's box we can't close?"
Societal Implications
The societal implications of CRISPR stretch far beyond science. First off, there's the issue of accessibility. Will this technology be available to everyone or only to those who can afford it? The divide could lead to a new form of inequality—one that's biological in nature. The potential to edit traits may turn into a breeding ground for elitism, where those with resources enhance their children’s abilities, creating a scenario reminiscent of speculative fiction.
Moreover, CRISPR raises questions about consent. When it comes to germline modifications, future generations wouldn't have the chance to agree or voice their opinions on the changes made to their genetic makeup. This creates ethical dilemmas surrounding human rights and autonomy. The ramifications of altering human genes are profound; they could change not just individuals but the very essence of what it means to be human.
Regulatory Frameworks
Given the weight of these ethical concerns, robust regulatory frameworks are essential. The current landscape of regulations regarding CRISPR is patchy at best. Although many countries have established guidelines, they vary significantly, which can complicate international research collaborations. An effective regulatory framework should aim to harmonize these guidelines while ensuring that ethical considerations are at the heart of scientific advancement.
Regulations must also account for the rapid pace of CRISPR development. Traditional regulations often lag behind innovation, creating potential loopholes that could be exploited. Keeping pace with scientific advancements while addressing public concerns around safety, efficacy, and ethics is crucial.
In short, CRISPR technology offers remarkable potential, but it’s vital to approach it with caution and a keen ethical lens. Balancing innovation and responsibility will be imperative as we forge ahead into a realm where gene editing may redefine medicine, agriculture, and human existence itself.
Future Directions in CRISPR Research
As we stand at the precipice of genetic innovation, it becomes crucial to explore the future pathways that CRISPR technology might traverse. The relevance of this discussion emanates not just from the advancements in precision editing but also from the broader implications for science, ethics, and society at large. The future of CRISPR can potentially reshape domains ranging from healthcare to agriculture and environmental sustainability.
Advancements in Precision and Efficiency
One of the most significant future directions for CRISPR research lies in enhancing its precision and efficiency. Current techniques can sometimes lead to off-target effects—unintended modifications to the DNA that can cause adverse side effects. The hunt is on for technologies that bolster the accuracy of CRISPR, such as improved guide RNA design and next-generation cas proteins. For example, researchers are looking at Cas12 and Cas13, which demonstrate unique properties that could minimize errors. The goal is to develop systems that can rectify themselves, essentially finding and correcting mistakes on the fly. This not only boosts the reliability of gene editing but also opens doors for therapeutic applications in a wide range of conditions, including genetic disorders and cancer.
- Increased Efficiency: Faster processing times for gene editing tasks will make it possible to conduct studies and treatments more rapidly than ever before.
- Lower Costs: Streamlined methods could lead to reduced expenses, making CRISPR accessible in various laboratories and clinics, especially in developing countries.
"The improvement in efficiency can translate to groundbreaking improvements in patient outcomes and crop yields alike."
Expanding CRISPR Applications
The potential applications for CRISPR are vast, and expanding these avenues is a vital future direction. Beyond the traditional realms of biomedicine and agriculture, innovative uses are emerging. Consider the potential of CRISPR in synthetic biology—a field where researchers are already crafting bacteria with tailored functionalities, like biofuel production or environmental cleanup. The capacity for localized pathogens to be edited for disease resistance also deserves mention, creating possibilities for agricultural shifts away from chemical pesticides.
Moreover, the tech doesn't stop at plants. For livestock, CRISPR could engineer traits such as increased disease resilience and enhanced nutrition profiles. The implications are profound:
- Food Security: Increasing global food demands could be met with modified crops that require less resource input.
- Sustainable Practices: Harnessing CRISPR’s ability to enhance biodiversity holds great promise for combating climate change.
Global Collaborative Efforts in CRISPR Research
To truly harness CRISPR's potential, global collaboration remains a cornerstone of future research. The complexity of genetic editing invites various stakeholders—governments, researchers, and even private industries—to join hands in understanding and utilizing CRISPR more effectively. International sharing of knowledge can fast-track the discovery of new applications and solutions.
Conferences, collaborative projects, and even online platforms encourage dialogues among experts worldwide. For instance, institutions can share findings on CRISPR implementations, lessons learned, and ethical considerations that arise.
- Open-Source Research: This allows for collective problems to be tackled more effectively, accelerating CRISPR advancements over competitive ventures.
- Cross-disciplinary Approaches: New ideas often come from different fields; integrating perspectives from computer science, ethics, and biology can result in groundbreaking insights.
In summary, as we look ahead, the future directions of CRISPR research hold vast promise for enhancing its precision and expanding its applications. The concerted efforts of the global scientific community will be pivotal in navigating the complexities ahead, ensuring that this powerful tool serves the greater good. The next frontier for CRISPR is not just technical—it's also deeply intertwined with ethics, collaboration, and the responsibility to wield this power wisely.
Finale: The Path Ahead for CRISPR Technology
As we stand on the precipice of a new era in biotechnology, the significance of CRISPR technology cannot be overstated. This tool promises to revolutionize the way we interact with genetic information. To fully appreciate its trajectory, one must consider not only the advancements made thus far but also the challenges and ethical considerations looming ahead.
Summary of Key Points
- Mechanisms of Gene Editing: CRISPR functions through a precise, targeted approach that markedly enhances gene editing capabilities compared to traditional methods. The Cas9 enzyme, guided by a specially designed RNA, seeks out specific DNA sequences to either cut or modify them.
- Diverse Applications: From addressing genetic disorders to enhancing crop resilience, the reach of CRISPR is expansive. Innovative tools such as CRISPR-Cas12 and CRISPR-based diagnostics underscore the versatility inherent within this technology.
- Ethical and Regulatory Frameworks: As with any groundbreaking technology, ethical dilemmas arise. The potential for unintended consequences in human health and ecological impact requires robust regulatory frameworks. There is an ongoing debate within scientific and public communities about the implications of germline editing, which could be passed to future generations.
- Future Innovations: The path ahead involves refining the precision of these techniques. Researchers are pursuing alternative molecular tools like CRISPR interference or CRISPR activation to provide even more nuanced control over gene expression.
"Regulation and innovation must walk hand in hand to ensure the responsible use of CRISPR technology."
The Role of CRISPR in Shaping Future Science
CRISPR is not just a tool; it's a catalyst for change in both fundamental research and applied sciences. Its influence can be seen across various fields:
- Medicine: CRISPR's potential to treat genetic diseases holds promise, bringing forward targeted therapies that could one day cure conditions previously thought untreatable.
- Agriculture: With global challenges such as food security and climate change dominating discussions, CRISPR provides innovative solutions. From creating disease-resistant crops to enhancing nutritional content, the agricultural applications are vast.
- Environmental Science: CRISPR could play a crucial role in addressing ecological issues, such as biodiversity loss and pollution remediation. Its capacity to edit the genomes of organisms may help restore ecosystems in jeopardy.
In essence, the future of CRISPR technology is bright, intertwined with the threads of scientific discovery and ethical contemplation. As we harness this powerful tool, the collaborative efforts among scholars, ethicists, and policymakers will determine the best paths forward. Promoting a transparent dialogue will help navigate the murky waters of genetic engineering, with the aim of ensuring that CRISPR technology serves humanity's best interests.