Comprehensive Guide to Growing Stem Cells
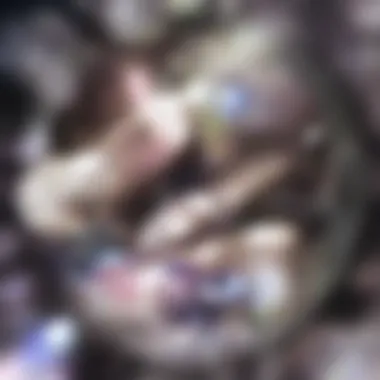
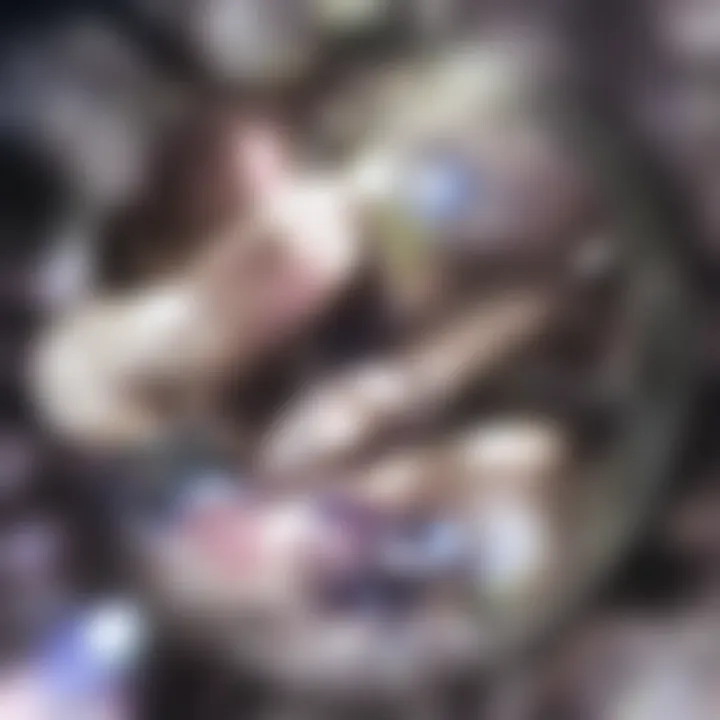
Intro
Stem cells have emerged as a pivotal focus within biomedical research and therapeutic applications. Their unique ability to differentiate into various cell types and renew themselves indefinitely makes them invaluable in fields like regenerative medicine, developmental biology, and pharmacology. This guide intends to unpack the nuances of stem cell growth, providing insight into both the theoretical and practical aspects associated with their cultivation and utilization.
Understanding the mechanisms that underpin stem cell growth involves delving into complex biological processes. From the pristine environments required for their optimal expansion to the sophisticated techniques employed for in vitro culture, this guide outlines each element necessary for the successful management of stem cells. Moreover, attention is given to the ethical considerations that arise from stem cell research, laying the groundwork for a holistic view of this significant area of study.
Research Context
Background Information
Stem cells are categorized into two primary types: embryonic and adult stem cells. Embryonic stem cells, derived from the inner cell mass of blastocysts, are pluripotent, which allows them to develop into any cell type in the body. In contrast, adult stem cells, also known as somatic stem cells, are multipotent and have a more limited ability to differentiate into cell types relevant to their tissue of origin. Recent advancements in stem cell research have raised the possibility of induced pluripotent stem cells (iPSCs), which are genetically reprogrammed adult cells that can behave like embryonic stem cells.
The environments in which stem cells thrive are multifaceted. Factors influencing stem cell growth include the availability of specific nutrients, the presence of growth factors, and the physical structure of the culture surface. The discovery of the stem cell niche, which represents the local microenvironment in which stem cells reside, has significantly enhanced our understanding of their growth and regulation.
Importance of the Study
Research in stem cell growth is not merely an academic pursuit; it holds profound implications for treatment strategies across various conditions. By cultivating stem cells in controlled environments, researchers can study diseases, develop therapeutic approaches, and even test pharmaceutical compounds. The potential to regenerate damaged tissues or organs through stem cell therapy continues to advance the field of medicine, highlighting the urgent need for in-depth exploration of stem cell biology.
Furthermore, ethical debates surrounding stem cell research emphasize the necessity for informed discussions about consent, potential exploitation, and the moral status of embryos. This guide does not shy away from addressing these challenges, aiming to provide a balanced perspective that marries scientific advancement with ethical responsibility.
“Stem cell research has the potential to change the course of medicine, offering treatments where there were none.”
In summary, this guide aims to furnish students, researchers, and professionals with a comprehensive understanding of stem cell growth. By addressing both the science and the ethical dimensions of this research, it seeks to foster knowledge that is critical in advancing the field and its applications into the future.
Understanding Stem Cells
Understanding stem cells is crucial in the realm of biological research and medicine. These remarkable cells hold the potential to transform various fields, from regenerative medicine to disease treatment. Stem cells serve as a foundation for developing therapies that can combat diseases such as cancer and neurodegenerative disorders.
This section aims to elaborate on what stem cells are, detailing their definition, types, and the unique characteristics that make them essential for scientific exploration.
Definition of Stem Cells
Stem cells are a unique class of cells characterized by their ability to self-renew and differentiate into various cell types. They do not have a specific function initially, which allows them the flexibility to develop into different tissues in the body. This property is what makes stem cells so valuable for research and therapy.
In a laboratory setting, stem cells can be isolated and cultured, providing a significant tool for studying development, disease, and therapeutic applications. Their pluripotent or multipotent nature allows them to contribute to understanding how different cells function and how diseases affect these functions.
Types of Stem Cells
Stem cells can be broadly categorized into three main types: embryonic stem cells, adult stem cells, and induced pluripotent stem cells. Each type possesses distinct traits that influence its utility in scientific and clinical settings.
Embryonic Stem Cells
Embryonic stem cells are derived from early-stage embryos. They are known for their pluripotency, which means they can develop into nearly any cell type in the body. This characteristic makes them particularly desirable for regenerative medicine.
The key aspect of embryonic stem cells is their ability to differentiate into a wide range of cell types, including neurons, cardiomyocytes, and tissue-specific cells. This adaptability makes them a powerful choice for producing tissues in the lab.
However, the use of embryonic stem cells raises ethical concerns due to the destruction of embryos in the extraction process. This limitation can hinder their acceptance and application in certain settings.
Adult Stem Cells
Adult stem cells, also known as somatic stem cells, are found in various tissues throughout the body. Unlike embryonic stem cells, they are typically multipotent, meaning they can only differentiate into a limited range of cell types related to their tissue of origin.
A notable characteristic of adult stem cells is their crucial role in repairing and maintaining tissues. For instance, hematopoietic stem cells found in bone marrow can give rise to all blood cell types. Due to their origin in adults, they are less controversial and often more readily accepted in clinical practices.
However, adult stem cells have a more limited differentiation potential compared to embryonic stem cells, which can restrict their applications in certain therapies.
Induced Pluripotent Stem Cells
Induced pluripotent stem cells, often referred to as iPSCs, are generated from adult somatic cells. Researchers can reprogram these cells using specific techniques to return them to a pluripotent state. This allows iPSCs to behave like embryonic stem cells, possessing the ability to differentiate into a range of cell types.
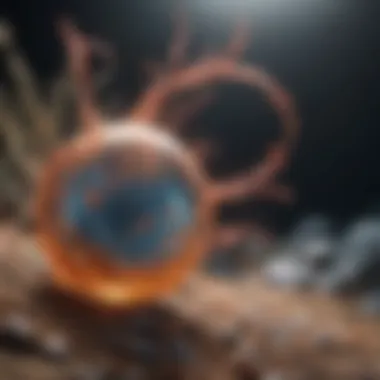
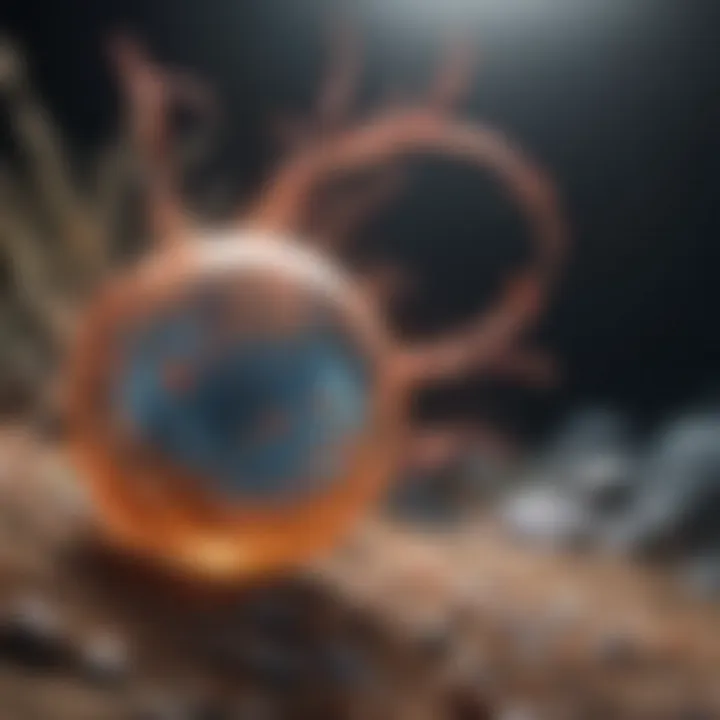
The significant advantage of iPSCs is that they avert the ethical issues associated with embryonic stem cells, as they do not require embryo destruction. Additionally, iPSCs can be derived from a patient's own cells, potentially minimizing the risk of immune rejection in therapy.
Despite their promise, the technology surrounding iPSCs is still developing, and it poses challenges such as tumorigenicity and the efficiency of the reprogramming process.
In summary, understanding the types of stem cells lays the groundwork for comprehending the wider implications of stem cell research. Each type comes with its own set of benefits and limitations, influencing the direction and application of research in this vital field.
The Biological Basis of Stem Cell Growth
Understanding the biological basis of stem cell growth is critical for any comprehensive guide on this subject. Stem cells have unique abilities that set them apart from other cell types. They can divide, self-renew, and differentiate into specialized cells. This versatility underscores their significant role in development, tissue repair, and therapeutic applications. The mechanisms that govern stem cell behavior are intricate and involve various biological processes, including the cell cycle and the stem cell niche.
Cell Cycle and Division
The cell cycle is fundamental to stem cell growth. It delineates the stages that a cell goes through to divide and proliferate. Stem cells can remain in a quiescent state or enter active division, which is crucial for maintaining their population. Their ability to transition between these states influences their availability and potency. For instance, when tissues are damaged, stem cells typically exit dormancy and begin to proliferate, thereby aiding in repair. This specific regulation ensures that stem cells can respond dynamically to physiological signals.
Stem Cell Niche
Definition and Importance
The stem cell niche refers to the specific microenvironment surrounding stem cells. It includes other cells, signaling molecules, and extracellular matrix components that interact with the stem cells. This niche plays a vital role in maintaining the characteristics of stem cells and controlling their fate. By providing necessary signals, the niche ensures that stem cells can either remain undifferentiated or commit to specific lineage paths when required.
The uniqueness of this concept lies in its dynamic nature. Factors in the niche can change in response to developmental cues or injury, making it a central player in stem cell biology. The versatility of the niche is both an advantage and a challenge; while it allows for flexibility, any disruption can lead to conditions like cancer or degenerative diseases.
Components of the Niche
The components of the stem cell niche are varied and complex. Critical elements include stromal cells, extracellular matrix proteins, and various growth factors. Each of these components contributes uniquely to the regulation of stem cells. For example, stromal cells provide support and essential signals that influence stem cell behavior.
One key feature of these components is their ability to collaborate in orchestrating stem cell activities. For instance, fibroblast growth factors (FGFs) are often found in niches and stimulate stem cell proliferation. However, their overabundance can lead to unwanted differentiation or tumorigenesis. Thus, while components of the niche are essential for stem cell growth, their regulation is equally important to prevent adverse outcomes.
The interplay between the stem cell niche and the intrinsic properties of stem cells determines the fate and functionality of stem cells, emphasizing the importance of this relationship in both health and disease.
In Vitro Methods for Growing Stem Cells
In vitro methods for growing stem cells are fundamental to the field of regenerative biology. These methods allow researchers to cultivate stem cells under controlled conditions, providing insights into their behavior, potential applications, and the biological mechanisms governing their functionality. The ability to grow these cells outside of a living organism not only facilitates the study of stem cells in a simplified environment but also enables various applications, including cell therapies and tissue engineering.
Culturing Techniques
Culturing techniques are crucial for maintaining the viability and functionality of stem cells during growth. Two primary approaches dominate this area: two-dimensional culture and three-dimensional culture.
Two-Dimensional Culture
Two-dimensional culture is the conventional method used in cell biology. It involves growing stem cells on a flat surface, typically in petri dishes or flasks. One significant advantage of this technique is its simplicity, which allows for straightforward monitoring and manipulation of the cells. This ease of access makes two-dimensional culture a popular choice among researchers.
However, there are limitations. Cells grown in two dimensions can behave differently than they would in vivo. They often lose some characteristics, such as their pluripotency and interact less with their environment. Limited spatial structure may also affect their growth patterns and functionality. Despite these challenges, two-dimensional culture remains a prevalent method in stem cell research due to its practicality and ease of use.
Three-Dimensional Culture
In contrast, three-dimensional culture attempts to more closely mimic the natural environment of stem cells. In this method, cells are grown in a structure that allows them to form aggregates or in scaffolds that support their growth in three dimensions. The key aspect of three-dimensional culture is its ability to replicate the cellular architecture seen in tissues, which can significantly influence the cells' behavior.
This technique promotes cell-cell and cell-matrix interactions, enhancing differentiation and functionality. While three-dimensional culture provides a more accurate representation of the in vivo environment, it can be more complex to set up and monitor. Moreover, it often requires specialized materials and techniques, which may complicate the growth process but yield richer data regarding stem cell behavior.
Growth Media Formulation
The success of in vitro growth methods relies heavily on growth media formulation. The selection of nutrients and supplements can significantly influence stem cell proliferation and differentiation.
Nutrient Requirements
Nutrient requirements encompass the essential components that promote growth and maintenance of stem cells. These include amino acids, vitamins, salts, and glucose, which serve as the building blocks for cellular activities. The type and concentration of these nutrients are critical for supporting cell viability. An appropriate formulation will ensure that cells can sustain metabolic processes and maintain their pluripotent state. This attention to nutrient balance also helps prevent issues like senescence, where cells lose their ability to divide effectively.
However, adjusting from one media to another or slightly changing concentrations can lead to variable results. Researchers must be meticulous in developing their nutrient formulations to achieve consistent outcomes.
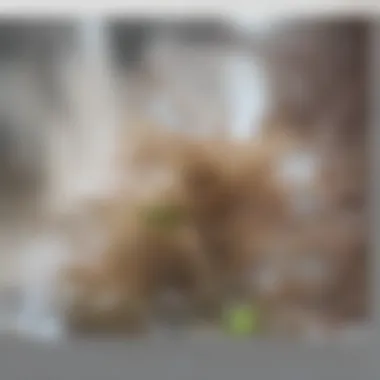
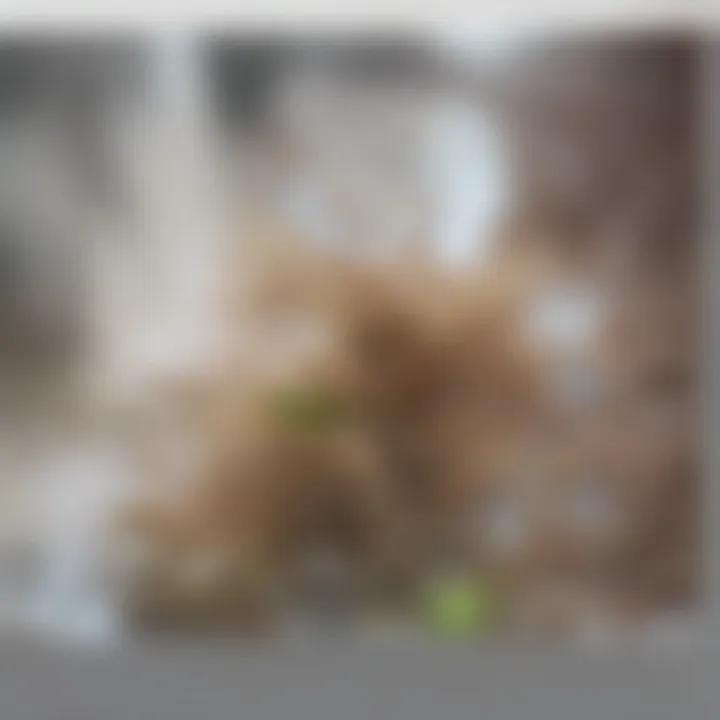
Supplements and Factors
Supplements and factors play a complementary role in growth media for stem cells. They include various signaling molecules, growth factors, and cytokines that facilitate cell proliferation and differentiation. A well-optimized media may contain entities like fibroblast growth factor (FGF) and epidermal growth factor (EGF), which are important for promoting stem cell growth.
The unique feature of adding these supplements is their ability to direct cell fate decisions. By manipulating media components, researchers can induce stem cells to differentiate into specific lineages. However, the precise composition of supplement mixtures must be carefully determined, as improper combinations can lead to undesired outcomes.
In summary, the formulation of growth media, along with the choice of culturing techniques, plays a critical role in the successful propagation of stem cells. Properly optimized methods support research activities and potential therapeutic applications.
These methods emerge as indispensable tools in the advancement of stem cell science, providing an avenue for deeper understanding and potential breakthroughs in regenerative medicine and biotechnology.
Characterization of Stem Cells
Characterization of stem cells is a crucial aspect of understanding their properties and potential applications. This process involves assessing both the morphology and function of stem cells to ascertain their identity and functionality. Proper characterization allows researchers to select appropriate stem cell types for specific applications, ensuring effective use in research and clinical settings.
Molecular attributes, growth patterns, and differentiation potentials characterize these cells. This understanding helps inform their use in therapies and further research. Characterizing stem cells effectively can enhance regenerative medicine outcomes and minimize the ethical implications associated with stem cell use.
Morphological Assessment
Morphological assessment is one of the first steps in characterizing stem cells. This process examines the physical characteristics of stem cells, such as shape, size, and arrangement. Through microscopy, researchers can observe differences in cell structure that indicate the stem cells’ type and state of growth.
Variations in morphology can signal differentiation stages or issues with culture conditions. For example, pluripotent stem cells often appear as tightly packed colonies, while differentiated cells may show more diverse shapes and sizes. Conducting consistent morphological assessments can help researchers maintain optimal growth conditions and ensure the desired cell types are cultivated.
Functional Assays
Functional assays are methods designed to evaluate the behavior of stem cells in various experimental contexts. These assessments provide insight into the cells’ capabilities, especially regarding pluripotency—the ability to differentiate into various cell types. Understanding these functions is vital for advancing therapeutic applications of stem cells.
Pluripotency Markers
Pluripotency markers are specific proteins and genes that indicate whether a stem cell maintains its ability to differentiate into multiple cell types. Examples include Oct4, Sox2, and Nanog. These markers provide a clear indication of a cell's undifferentiated state. In practice, confirming the presence of pluripotency markers is essential in experiments to ensure that cells have not unintentionally differentiated.
The benefit of using these markers is their high specificity and sensitivity, making them reliable indicators in both research and clinical applications. However, challenges exist in maintaining these markers' expression, especially under varying culture conditions. Understanding their behavior is critical in ensuring the stem cells' integrity throughout the research.
Lineage Tracing
Lineage tracing is another vital method of characterization that tracks the developmental path of stem cells as they differentiate. This approach typically involves genetic labeling techniques to mark the initial cells and observe their subsequent fate.
The key characteristic of lineage tracing is its ability to provide a dynamic view of the differentiation process. This method is increasingly popular because it offers deep insights into how stem cells contribute to various tissues and organs over time. Understanding these developmental pathways is important for therapeutic strategies aiming to regenerate specific tissues.
Despite its advantages, lineage tracing can present technical challenges, including the complexity of labeling and analyzing cell populations over time. Ultimately, lineage tracing significantly enriches our understanding of stem cell dynamics, connecting basic research with therapeutic applications.
Applications of Stem Cell Growth
The applications of stem cell growth represent a critical area of research and development in modern science. Understanding how stem cells can be applied to various fields enables researchers and practitioners to explore innovative solutions for complex medical problems. Stem cell research continues to uncover potential breakthroughs in disease treatment, regenerative therapies, as well as advancements in drug development and tissue engineering.
Regenerative Medicine
Regenerative medicine is one of the most profound applications of stem cell growth. This field focuses on repairing or replacing damaged or diseased tissues and organs by harnessing the unique properties of stem cells. For example, stem cells derived from various sources can differentiate into specific cell types needed for tissue repair. Such capabilities make them invaluable in addressing conditions like heart disease, spinal cord injuries, and neurodegenerative disorders.
In clinical settings, advancements have already been made. Mesenchymal stem cells, for instance, have been used in trials to regenerate cartilage in osteoarthritis. Moreover, hematopoietic stem cell transplants are established procedures for treating blood disorders. The potential benefits of regenerative medicine using stem cells extend beyond immediate healing. They also include long-term improvements in patients' quality of life.
Disease Modeling and Drug Testing
Another significant application of stem cell growth is in disease modeling and drug testing. By using induced pluripotent stem cells (iPSCs) derived from patients, researchers can create cellular models of various diseases. These models are instrumental in understanding disease mechanisms at a cellular level. They allow researchers to observe how diseases progress and interact with potential treatments.
Drug testing also benefits from the use of stem cells. Traditional drug testing often relies on animal models, which do not always accurately predict human reactions. By testing drugs on human-derived stem cell models, researchers can enhance the predictive value of preclinical testing and streamline the development of new therapies. This approach could potentially reduce the time and costs associated with bringing new drugs to market.
Tissue Engineering
Tissue engineering is an evolving field that combines principles from biology and engineering to develop biological substitutes that restore, maintain, or improve tissue function. Stem cells play a crucial role here, as they can be guided to form various tissue types through a combination of scaffolding materials and growth factors. This integration allows researchers to create tissues that mimic native structures and functions.
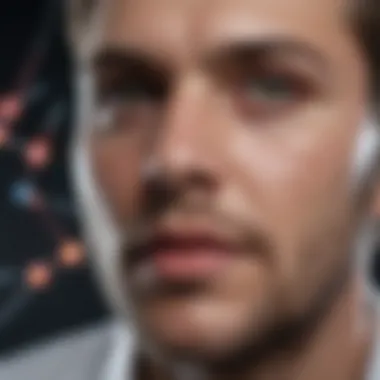
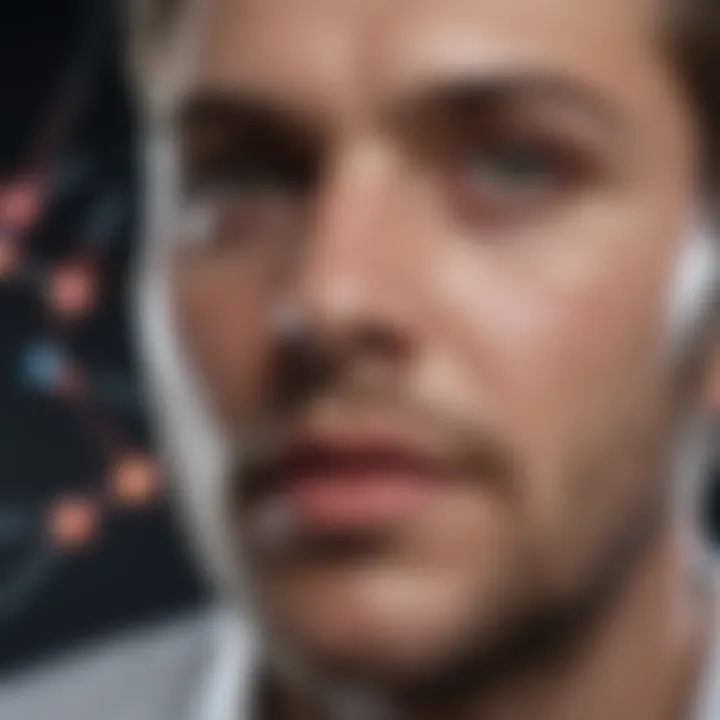
Applications in tissue engineering are vast. For example, bioengineered skin grafts composed of stem cells are already in use for treating burn patients and other skin injuries. Additionally, progress is being made in engineering heart tissues and cartilage, which hold promise for treating degenerative joint diseases and cardiovascular conditions. The three-pronged approach of stem cells, scaffolding, and biochemical signals creates a robust platform for advancing tissue repair and regeneration.
In summary, the applications of stem cell growth reflect the versatility and potential that stem cells hold for advancing medical science. Regenerative medicine, disease modeling, and tissue engineering are just a few examples of how ongoing research can lead to innovative therapies and transformative healthcare solutions.
Challenges in Stem Cell Growth
Understanding the challenges in stem cell growth is crucial for anyone involved in stem cell research or application. These challenges can impede progress in regenerative medicine, disease modeling, and other scientific endeavors. Addressing these issues is essential to effectively harness the potential of stem cells, which could significantly advance healthcare.
Technical Limitations
One of the primary challenges faced in stem cell growth includes technical limitations associated with culturing methodologies. For instance, achieving the correct environment that mimics the in vivo conditions of niche cells is difficult. This includes an appropriate balance of nutrients, oxygen, and physical space. Researchers often encounter issues with maintaining stem cell pluripotency during long-term cultures, which can lead to differentiation or senescence.
In addition, scalability poses a significant barrier. Many in vitro techniques produce limited quantities of stem cells, which may not suffice for therapeutic applications. Inadequate methodologies may result in inadequate cell populations to support research or treatment initiatives. Furthermore, the variability between cell lines can complicate reproducibility in experimental settings, making it hard to standardize outcomes across studies.
To overcome these limitations, researchers are exploring advanced culturing techniques such as bioreactors that provide controlled environments. Yet, these technologies are still evolving and require further refinement to realize their full potential in stem cell proliferation.
Ethical Considerations
Ethical concerns surrounding stem cell research cannot be overlooked. The main issue arises with the use of embryonic stem cells, which requires the destruction of human embryos. This practice raises profound moral questions and has sparked considerable debate within societies. Different regions have varied regulations and ethical guidelines regarding the sourcing of stem cells.
Moreover, issues related to consent are essential, particularly when deriving stem cells from humans. Researchers must ensure that donors are fully informed and consent freely. This ethical responsibility extends to the use and disposal of cells in research as well.
Public opinion on stem cell research can also influence funding and policy decisions. Advocacy for responsible research that prioritizes ethical considerations is crucial for moving forward. As technology advances and methods like induced pluripotent stem cells become more popular, the ethical landscape must adapt. By navigating these ethical waters thoughtfully, researchers can find a balance that respects human life while promoting scientific exploration.
"Navigating the ethical landscape of stem cell research is as imperative as overcoming the technical challenges. Both dimensions must coalesce to unlock the full potential of stem cell science."
In summary, overcoming technical limitations while addressing ethical concerns form the cornerstone of effective stem cell growth. Engaging with both areas can pave the way for advancements in stem cell therapies and applications.
Future Directions in Stem Cell Research
The field of stem cell research is constantly evolving. With advancements in technology and increasing understanding of cellular biology, the potential for stem cells expands. Future directions in this area are critical as they shape new therapeutic approaches and enhance our understanding of human biology. Exploring these future pathways allows researchers and clinicians to visualize what is possible in regenerative medicine, disease treatment, and biological exploration.
Emerging Technologies
Emerging technologies are revolutionizing how scientists approach stem cell research. Key advancements include CRISPR gene editing, organ-on-a-chip systems, and artificial intelligence algorithms.
- CRISPR Gene Editing: This technology enables precise modifications to the genome. Researchers can correct genetic defects in stem cells, potentially leading to therapies for hereditary diseases.
- Organs-on-Chips: These microengineered devices simulate human organ systems. They allow for the study of stem cell differentiation and drug response in a controlled environment, enhancing our understanding of organ development.
- Artificial Intelligence: AI aids in analyzing large datasets from stem cell experiments. It can predict cell behavior and optimize culture conditions, leading to more efficient stem cell growth strategies.
Such technologies not only offer new methods but also address existing challenges. Their integration into stem cell research will likely increase the efficiency and effectiveness of cell-based therapies.
Potential Clinical Applications
The clinical applications of advanced stem cell technologies are immense and far-reaching. These potential uses can enhance personalized medicine significantly.
- Regenerative Therapies: Stem cells have the power to regenerate damaged tissues in conditions such as heart disease or spinal injuries. Targeted approaches using gene editing could lead to even more effective treatments.
- Cancer Treatment: Stem cells can be engineered to attack cancer cells specifically. This precision reduces collateral damage to healthy tissues, allowing for safer treatment options.
- Drug Development: Stem cells can model diseases, providing valuable platforms for testing new drugs. This leads to more reliable results, reducing reliance on animal models.
- Autoimmune Diseases: Research suggests that stem cells can modulate the immune system. This opens doors for new therapies in conditions like multiple sclerosis and rheumatoid arthritis.
Through these applications, the impact of stem cell research may extend from lab settings into everyday clinical practices. As research progresses, the hope is to translate findings into effective treatments, improving patient outcomes.
"The future of stem cell research holds transformative potential, paving the way for innovative treatments and deeper insights into cellular functions."
End
The conclusion of this article encapsulates the essential insights and implications of stem cell growth. Understanding how to effectively cultivate and manipulate stem cells is crucial not merely for academic enrichment, but for its profound applications in medicine and research. This section aims to reiterate the main points while emphasizing the forward-looking perspective that stem cell research offers.
First, the cultivation of stem cells has the potential to revolutionize regenerative medicine. With the ability to regenerate damaged tissues and organs, stem cells present a groundbreaking avenue for treating a myriad of diseases. Therapies derived from stem cells can offer patients alternatives to traditional treatments, thereby transforming healthcare modalities.
Moreover, as we explored various growing methodologies, it became clear that in vitro culture techniques are pivotal. These methodologies allow for a controlled environment where stem cells can proliferate and differentiate under monitored conditions. This not only enhances understanding of cell behaviors but also optimizes conditions for maximum yield.
Additionally, addressing the challenges associated with stem cell growth—including technical limitations and ethical considerations—is imperative. Researchers must navigate these obstacles to advance the field responsibly. Ethical discussions ensure that research aligns with societal values and safety protocols, which is vital for public trust and acceptance.
Finally, as we look ahead, the future of stem cell research appears promising. Emerging technologies and novel applications continue to evolve, pushing the boundaries of what is possible. As scientists develop new techniques, the landscape of medical solutions becomes more innovative and impactful.
"The exploration of stem cell growth is not just a scientific endeavor; it's a journey towards unlocking the fundamental mysteries of life itself."
In essence, as this guide illustrates, stem cell growth stands at the confluence of scientific innovation and ethical responsibility. The knowledge acquired through such investigations will undoubtedly shape future medical practices and enhance our approach to human health.