Exploring Hydrate Formulas: Chemistry and Applications
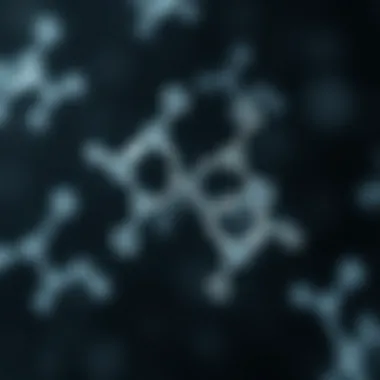
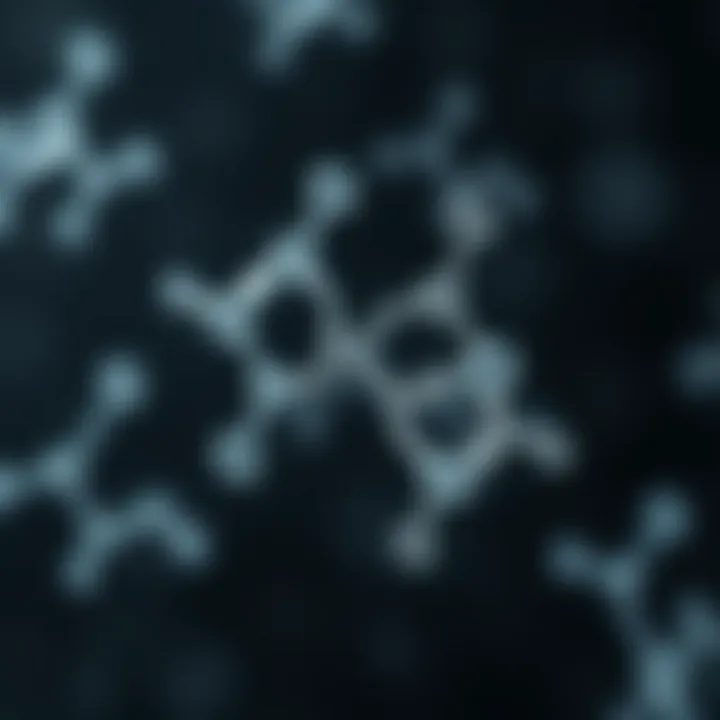
Intro
Hydrate formulas may seem like mere formulas scribbled in a chemistry textbook, but these intricate representations hold more significance than one might think. At first glance, one might brush them off as just another topic to skim through in a science class, yet they intersect with various aspects of our lives, from the pharmaceuticals we consume to the construction materials we use. This article aims to delve deeper into the veil surrounding hydrate formulas, peeling back layers to reveal their importance across numerous domains.
Distinctions between hydrates and anhydrous compounds can often feel like splitting hairs. However, understanding this difference is crucial. Hydrates are compounds that have a specific number of water molecules integrated into their crystalline structure, impacting their properties and behavior. For example, the presence of water in crystalline structures can change how substances like copper(II) sulfate behave in various chemical reactions.
Just as a sponge retains water, hydrates have unique traits attributed to this water content. Addressing the implications of these compounds helps bridge the gap between abstract concepts and their real-life applications. Moreover, the ongoing research into hydrates not only sheds light on basic scientific principles but also aligns with innovations in industries such as materials science and biochemistry.
As we navigate through the depths of this study, we will uncover how hydrates are synthesized, their roles in biological systems, and much more. Buckle up for an in-depth journey to grasp the intricacies of hydrate formulas, laying the groundwork for a better understanding of their functions in our world.
Preface to Hydrate Formulas
Hydrate formulas represent a fascinating intersection of chemistry and practical applications, playing a crucial role in various fields such as biology, environmental science, and material technology. Understanding these formulas is more than just an academic pursuit; it is fundamental to grasping how molecules interact with water and the impact of such interactions on the world around us. By exploring the significance of hydrates, one can appreciate their importance in sustaining life, driving industrial processes, and contributing to technological advancements.
Definition and Significance
Hydrates are compounds that include water molecules as an integral part of their structure. The water can be found in large quantities, and understanding how these hydrates function is crucial. For instance, many minerals exist in hydrate forms, and their stability can be influenced by environmental conditions. This characteristic has broad implications, particularly in earth sciences and mining.
Moreover, hydrates serve important roles in biological systems, influencing cellular hydration processes and metabolism. In the materials sector, the stability and properties of hydrates can determine the performance of construction materials and other industrial products. Consequently, recognizing their definition and significance helps bounce the conversation from mere theory to real-world implications.
Historical Context
The journey into the realm of hydrate chemistry began centuries ago, with early scientists noting the relationship between compounds and water. One notable figure is the chemist Louis Jacques Thรฉnard, who, in the early 19th century, conducted experiments that highlighted the role of water in crystal structures. This laid the groundwork for subsequent studies that explored both the chemical and physical properties of hydrates.
Over time, researchers have built upon these findings, leading to significant advancements in our understanding of hydrates, such as their formation mechanisms and stability factors. In recent decades, interest has surged due to the impact hydrates have on climate research, notably concerning methane hydrates in oceans โ a compound linked to greenhouse gas emissions.
Marking milestones through various eras of discovery illustrates how our comprehension of hydrates has evolved and points to a consensus that further study could yield insights with even broader implications, especially in the fields of sustainability and resource management.
Chemical Composition of Hydrates
Hydrates, compounds that consist of water and other chemical species, serve as an essential link between the molecular world and practical applications across a myriad of fields. The study of their chemical composition reveals not only the structure and bonding within these compounds but also their relevance in biological systems, environmental science, and material properties. Understanding the intricate balance of variables that dictate their formation and behavior is key for scholars and practitioners alike.
Molecular Structure
At the heart of hydrate chemistry is the molecular structure, which fundamentally dictates the properties and behavior of hydrates. When we examine this structure, we find that water molecules are integrated into the lattice of other compounds, often forming coordination complexes. For instance, the mineral blue vitriol, commonly known as copper (II) sulfate pentahydrate, has the formula CuSOโยท5HโO. Here, five water molecules are coordinated to each copper sulfate unit, creating a unique arrangement.
The distinctive aspect of these structures lies in the hydrogen bonds between water molecules and the host compound. These bonds can vary in strength based on environmental conditions such as temperature and pressure, making the study of hydrates an interesting field. For example, when subjected to heat, hydrates can lose their water content through a process called dehydration, which leads to changes in physical properties, color, and solubility.
"The interplay of molecular structure and environmental conditions is vital in determining the stability and applications of hydrates."
Understanding the molecular geometry and the hydrogen bonding networks offers insight into how hydrates behave under specific conditions. It plays a crucial role when considering applications in catalysis, energy storage, and even pharmaceuticals, where controlled release of water can be beneficial.
Common Examples
Common examples of hydrates not only illustrate the diversity of these compounds but also underscore their extensive usefulness:
- Epsom Salt (MgSOโยท7HโO): This compound is well-recognized for its solubility and hydration properties, often employed in agriculture and medicine.
- Boric Acid Pentahydrate (HโBOโยท5HโO): Used in pest control and as an antiseptic, this hydrate showcases how the presence of water in its structure enhances its reactivity.
- Calcium Sulfate Dihydrate (CaSOโยท2HโO): Known as gypsum, itโs prevalent in construction, emphasizing the importance of hydrates in industry.
These examples illustrate how the chemical composition affects functionalities. Whether itโs relating to the thermal properties of materials or their ability to store moisture for biological processes, these compounds demonstrate that the molecular formula reveals more than just chemical makeup.
In sum, the chemical composition of hydrates shapes their identity and functionality across various domains. From practical uses in construction to implications in biological hydration processes, a firm grasp on the molecular structure and examples provides a solid foundation for understanding hydrates in their entirety.
Types of Hydrates
Understanding the types of hydrates is crucial for grasping their functions and applications in various scientific fields. Hydrates can be classified primarily into two categories: simple and complex hydrates. Each type possesses its unique structure, properties, and implications for use in both natural and industrial contexts. Delving into these types reveals not only their individual characteristics but also how they interconnect within broader chemical processes.
Simple Hydrates
Simple hydrates, as the name suggests, are relatively straightforward in their makeup. They typically consist of a stable compound combined with water molecules integrated into their crystalline structure. A prime example of a simple hydrate is CuSOโยท5HโO, commonly known as copper(II) sulfate pentahydrate. In this compound, five water molecules are coordinated to one copper sulfate unit, illustrating how water molecules can enhance the stability and solubility of certain salts.
The significance of simple hydrates canโt be overstated. Many substances in everyday life, such as salts and minerals, are simple hydrates. They often play vital roles in sectors ranging from agriculture to pharmaceuticals. When exposed to heat, simple hydrates tend to lose their water component, transforming into anhydrous forms, which can thus be essential in controlling moisture levels in various applications. This ability to release and absorb water helps regulate and stabilize chemical processes, making them invaluable in industrial and laboratory settings.
So, when studying simple hydrates, one key point to consider is their phase changes and how these impact their use in different scenariosโbe it in hydration states in the environment or the applications in specialized material synthesis.
Complex Hydrates
Complex hydrates, on the other hand, represent a more intricate layer of hydrate chemistry. These forms often consist of metal complexes where the water molecules play a vital role not merely as a component but as part of a larger coordination entity. For instance, the hydrate [Co(HโO)โ]Clโ represents cobalt(II) chloride hexahydrate, where six water molecules are bonded to a cobalt ion, underscoring an important aspect of coordination chemistry.
The behaviors of complex hydrates are influenced by multiple factors, such as the type of metal ion involved and the ligands that are present. This leads to a broader variety of properties; some complex hydrates can form in response to specific environmental conditions, allowing for versatility across chemical applications. This adaptability is essential in sectors like catalysis, where understanding how these hydrates react under various conditions can lead to novel reactions and products.
Moreover, complex hydrates can often display intricate phase behaviors that can be both puzzling and fascinating. Different hydration states can offer unique solubility or stability characteristics, vital for fields such as materials science or drug formulation. Keeping this in mind, complexities in hydration states add depth to our understanding and utilization of hydrates, making them an area ripe for continued research.
"Understanding the types of hydrates shapes our approach to their practical applications and enhances our knowledge of material properties and processes."
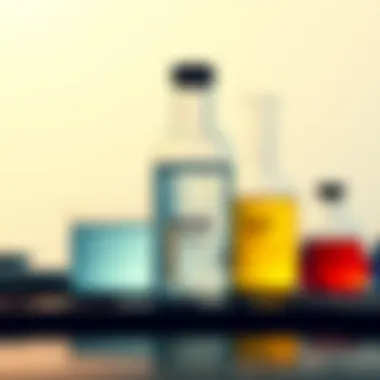
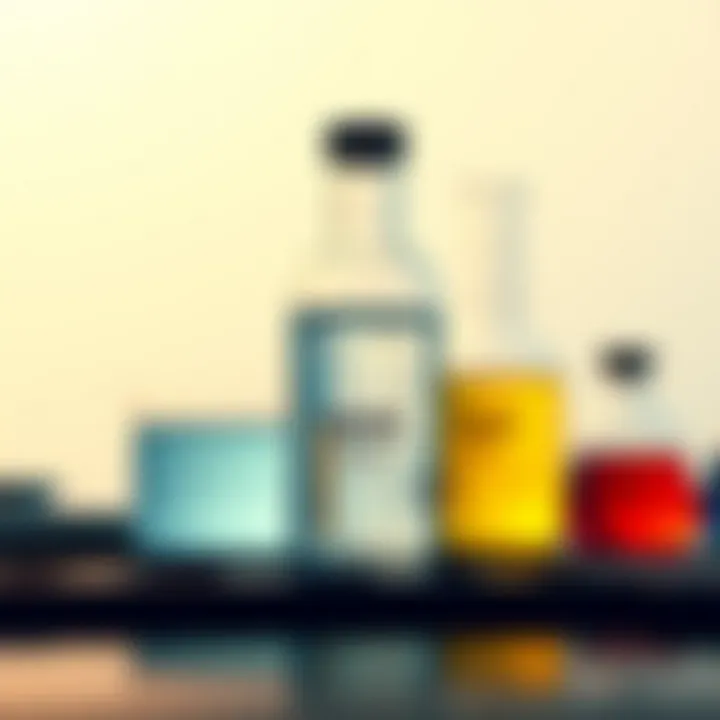
By examining both simple and complex hydrates, we appreciate their diversity and the essential roles they play in various disciplines. This distinction not only aids in academic exploration but also carries significant implications for innovative applications and research initiatives.
Formation and Stability of Hydrates
Understanding how hydrates form and remain stable is crucial in various disciplines, from chemistry to environmental science. The study of hydrate formation not only informs us about the conditions and processes that lead to the creation of these compounds but also opens up discussions regarding their usage and relevance in different industries. Moreover, grasping the factors that influence the stability of hydrates aids researchers and practitioners in predicting their behavior in natural and experimental settings, making this topic both practical and theoretically significant.
Mechanisms of Formation
The mechanisms of formation for hydrates are primarily based on the interactions between water molecules and solutes. This process can vary greatly depending on the conditions surrounding it. In general, hydrates form when water molecules become integrated into the crystalline structures of other compounds. This usually happens under specific temperature and pressure conditions.
For instance, when you have a salt, like sodium chloride, dissolving in water, the water molecules can form a structured arrangement around the ions. This organization leads to the creation of a hydrate, where water molecules become part of the lattice structure of the salt. Notably, the formation of hydrates can proceed through multiple pathways, including:
- Evaporation: When water evaporates and conditions allow for solute deposition alongside water.
- Cooling: When a saturated solution cools, leading to the precipitation of hydrates.
- Crystallization: The direct interaction of water vapor with crystalline solids at specific temperatures.
Thus, one could say that the formation of hydrates is akin to a dance between molecules, where temperature, pressure, and concentration dictate the rhythm of the steps taken.
Factors Affecting Stability
Several factors can affect the stability of hydrates, and understanding these can be beneficial for both theoretical research and practical applications. Some key factors include:
- Temperature: Increasing temperatures often lead to the breakdown of hydrate structures, causing them to lose water content and revert to their anhydrous forms.
- Pressure: Similarly, pressure variations can cause shifts in stability, especially in geological settings where hydrates naturally occur.
- Chemical Composition: The nature of the solute can greatly influence hydrate stability. For example, some salts form hydrates that are quite stable, while others may only do so under very specific conditions.
- Water Activity: The amount of unbound water in a system influences how well a hydrate will form or remain stable.
"Hydrates are not just mere footnotes in chemistries. Their formation and stability reflects a broader understanding of substance interactions in various environments."
Role of Hydrates in Biological Systems
Hydrates play a pivotal role in the intricate dance of life. At their core, these chemical structures house not only water but also essential nutrients and ions, forming the backbone of many biological processes. Their significance extends from cellular hydration to metabolic pathways, serving as crucial players in our bodyโs drama. Understanding how hydrates function can illuminate their benefits and implications in various biological contexts.
Hydration Processes in Cells
Cells, the building blocks of life, rely heavily on hydration. Every cell maintains a balance between fluid and solute, an equilibrium that hydrates ensure is met. The cell membrane allows water and solutes through, creating a semi-permeable barrier.
In simple terms, think of this as a bustling city; the membranes act like gates that control who goes in and out, keeping the environment stable. Without hydrates, cells would struggle to maintain their shape and functionality. Water molecules, being polar, interact with various cellular components, setting up a biochemical landscape crucial for activities such as nutrient absorption and waste removal.
"The proper hydration of cells is fundamental to all metabolic reactions, and without it, life as we know it would be impossible."
The hydration process often involves cytoplasmic hydration, where water molecules surround and interact with proteins, lipids, and other macromolecules. This is where hydration shells come into play; they help stabilize these molecules, allowing for the appropriate biochemical reactions to unfold. Understanding these hydration processes can aid in countless fieldsโfrom health sciences to biotechnologyโgiving insights into cellular functions and drug delivery systems.
Impact on Metabolism
Metabolism, the grand tapestry of biological reactions converting food into energy, is heavily influenced by the presence of hydrates. Hydration is crucial for enzymatic function, which governs the rate of metabolic reactions. Enzymes, the catalysts that drive these reactions, require an optimal aqueous environment to operate. Without this, processes like glycolysis and the Krebs cycle could grind to a halt.
Furthermore, the concept of osmotic balance is intertwined with metabolism. Water molecules facilitate the movement of nutrients in and out of cells, making it possible for metabolic pathways to access substrates when needed. The implications can be profound:
- Dehydration can slow down metabolic rates, leading to fatigue and diminished cognitive functions.
- Hydration boosts energy levels and improves overall cellular performance.
Thus, the impact of hydrates on metabolism not only affects basic biological functions but also influences our health and well-being. Researchers are increasingly focused on understanding the role of hydrates in metabolic disorders, as the interplay between hydration and metabolism opens new avenues for therapeutic strategies.
Hydrates in Environmental Science
Hydrates play a pivotal role in environmental science, with their impact sprawling across various facets of geochemistry and climate dynamics. Understanding their significance not only enriches our awareness of natural processes but also enhances the approach to tackle environmental challenges. Hydrates can be seen almost as natureโs hidden treasures, playing a crucial role in storing and disseminating crucial resources like water and energy in various ecosystems.
Importance in Geochemistry
Hydrates are central to geochemical processes, particularly in marine and geological environments. They are often formed in regions where high pressure and low temperature exist, such as the deep sea or polar regions. This process is not just a chemical curiosity; it has practical implications. For instance, the presence of hydrates in sediments can affect the stability of the seafloor, influencing underwater construction projects and natural resource extraction.
Moreover, hydrates act as agents for storage and transportation of natural gas. Methane hydrates, for example, contain vast amounts of methane trapped in ice-like structures, representing a potential energy source that, if harnessed responsibly, could contribute to energy needs without massive land disruption.
- Energy storage: Methane hydrates hold potential as clean energy carriers.
- Geological stability: Their presence alters the physical properties of sediments, impacting construction and exploration activities.
Role in Climate Change
The relevance of hydrates extends into the realm of climate science. The melting of hydrate deposits due to rising global temperatures can release significant amounts of methaneโa greenhouse gas exponentially more potent than carbon dioxide over short periods. This phenomenon presents a dual challenge: not only does it contribute to climate change, but it also poses risks to ecosystems and human infrastructures.
- Greenhouse gas emissions: As global temperatures rise, the destabilization of hydrates could lead to increased methane emissions, amplifying climate change effects.
- Feedback mechanisms: The interaction between hydrates and climate systems underscores the complex interdependencies that scientists must explore in their research efforts.
"Examining hydrates offers insights into both the potential solutions and significant challenges posed by climate change, emphasizing their dual role in our planetโs future."
The ongoing research into hydrate behavior under varying environmental conditions emphasizes their crucial position in studies of carbon cycling and overall ecological health. Addressing the effects of hydrates in environmental contexts provides a pathway to develop sustainable practices and mitigate against adverse climate impacts. Understanding their roles also aids policymakers in crafting informed strategies designed to balance energy needs with environmental considerations.
Hydrates in Material Science
The exploration of hydrates within material science provides a unique lens to understand their vital role in various applications and innovations. These compounds, which incorporate water into their structure, are not just a niche topic; they have implications in fields ranging from construction to electronics. The discernment of their properties expands the potential for both existing and future materials, promising advancements that could significantly enhance performance and sustainability.
Thermal Properties
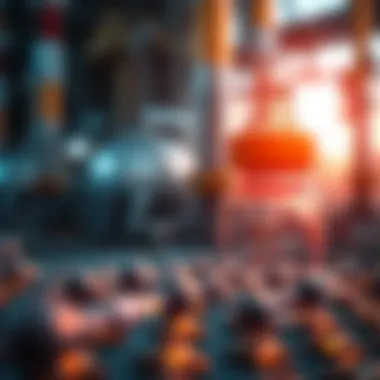
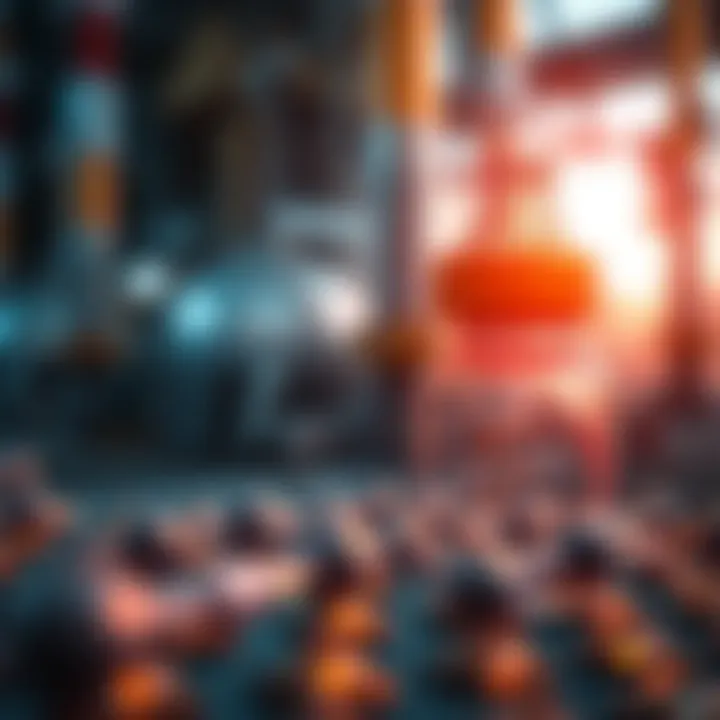
Hydrates are known to possess intriguing thermal properties that make them beneficial in various sectors. Their ability to absorb and release heat during phase changes can be used effectively in thermal energy storage systems. For instance, consider the use of calcium sulfate dihydrate in construction materials. When mixed with gypsum, it not only helps in regulating temperature but also enhances the durability and longevity of structures. This property is known as latent heat storage, where hydrates can maintain a stable temperature in buildings, ultimately leading to reduced energy consumption.
Moreover, the thermal stability of certain hydrates plays a crucial role in applications that require resistance to extreme temperatures. This is particularly important in manufacturing processes, where hydration compounds can influence the thermal management of sensitive materials. By understanding the specific thermal behaviors of hydrates, scientists and engineers can formulate materials with tailored thermal properties for enhanced performance.
Mechanical Properties
The mechanical properties of hydrates are equally essential, influencing their effectiveness in various applications. Hydrates can contribute to the strength and resilience of composite materials. For example, sodium acetate trihydrate is used in phase change materials that are incorporated into building materials. These materials not only offer structural support but also possess the capacity to respond dynamically to changes in temperature, which can improve energy efficiency and decrease heating costs.
The addition of hydrates to material formulations can yield significant improvements in compression and tensile strength, thanks to the structural integrity they confer. For instance, when aluminum hydroxide is combined with other polymers, it enhances the mechanical strength of plastics while also imparting flame-retardant properties. This characteristic is particularly beneficial in industries where safety and performance are paramount.
"The interplay between hydration and material strength opens avenues for innovative applications, driving research toward multi-functional materials that serve diverse purposes."
In summary, the importance of hydrates in material science cannot be overstated. Their thermal and mechanical properties not only enhance existing materials but also pave the way for novel applications that can address contemporary engineering challenges. As researchers continue to delve into the characteristics of hydrates, we may yet witness transformative developments that redefine how materials are designed and utilized in various industries.
Manufacturing and Synthesis of Hydrates
The manufacturing and synthesis of hydrates is a cornerstone of both scientific inquiry and industrial application. Understanding how hydrates are created offers vital insights into their properties, behaviors, and potential innovations. In a world that increasingly seeks efficiency and sustainability, mastering synthesis methods provides pathways to optimize material performance, reduce costs, and capitalize on unique chemical attributes of hydrates.
When considering manufacturing processes, one must appreciate both the economic and environmental factors involved. Effective synthesis can yield high-purity hydrates that are crucial for various applications, minimizing waste and promoting sustainability. This section will traverse the diverse landscape of synthesis methods that researchers and industries employ to obtain hydrates while exploring the inherent benefits and considerations of each approach.
Common Synthesis Methods
Hydrate formations can occur through several synthesis methods, each adaptable to specific conditions and types of hydrates. Here are the most prevalent techniques:
- Cool fusion method: For certain clathrate hydrates, this technique involves cooling gas or vapor mixtures in aqueous solutions, inducing hydrate crystallization at lower temperatures. Itโs effective for producing hydrates with specific stoichiometries.
- Pressure application: Using high pressure, particularly in gas hydrates, allows water and gas molecules to interlock into a structured lattice. This method highlights the importance of thermodynamics in hydrate formation, making it a viable option for capturing natural gases like methane.
- Solvent-assisted synthesis: Here, other solvents besides water facilitate hydrate growth. This method can help in creating hydrates with tailored properties.
"The synthesis of hydrates must consider not only the desired product but also the implications for scalability and environmental footprint."
- Hydrothermal synthesis: In this method, high-temperature and high-pressure environments foster the growth of hydrates. While efficient, the energy costs can be a concern.
Employing these varied techniques enables manufacturers to tailor the properties of hydrates, enhancing their applicability across industries ranging from energy to pharmaceuticals.
Industrial Applications
Hydrates have cemented their role in numerous industrial sectors due to their unique properties and behaviors. Here are a few notable applications:
- Energy sector: Hydrate deposits present a potential source of clean energy. Methane hydrates, for instance, can be tapped as a natural gas resource, offering a more environmentally friendly alternative to traditional fossil fuels.
- Chemical storage and transport: Hydrates can act as a medium for storing gases. For example, the encapsulation of gases in hydrates helps in reducing pollutants during transport. This characteristic is increasingly important as industries move towards greener practices.
- Material sciences: Hydrates are utilized in various materials to modify thermal and mechanical properties. Their ability to absorb and release substantial amounts of heat makes them ideal for applications in temperature regulation systems.
- Environmental remediation: Some hydrates can be engineered for environmental cleanup processes, such as the removal of contaminants from water sources.
By understanding the manufacturing and synthesis of hydrates, organizations stand to benefit significantly. This growing field not only illustrates the versatility and significance of hydrates but also beckons a future where their careful manipulation could lead to remarkable advancements across different sectors.
Analysis and Characterization Techniques
When it comes to studying hydrates, the crux lies in systematically understanding their composition, structure, and behavior under various conditions. Analysis and characterization techniques are pivotal in achieving this understanding. They allow scientists to discern the molecular intricacies of hydrates and assess how these compounds interact with their environment. By employing robust methods, researchers can accurately determine properties that drive both practical applications and theoretical advancements.
Spectroscopic Methods
Spectroscopic methods serve as the bedrock of many analytical techniques used to investigate hydrates. These methods utilize the interaction of electromagnetic radiation with molecules to provide insight into their structural nuances. The precision and versatility of spectroscopy make it an essential tool in hydrate analysis.
- Infrared (IR) Spectroscopy: This technique detects molecular vibrations and can identify specific functional groups within hydrate compounds. It's fundamental for understanding how water molecules are integrated into crystal lattices. A sharp peak in an IR spectrum might indicate the presence of water in a hydrate and give clues about its bonding nature.
- Nuclear Magnetic Resonance (NMR): NMR spectroscopy exploits the magnetic properties of certain nuclei. It can offer detailed information about the local environment of atoms in hydrates, particularly revealing the dynamics of water molecules and how they participate in hydrogen bonding. This can often lead to insights regarding the stability of hydrates under varying temperature and pressure.
- X-ray Diffraction (XRD): Primarily used for determining crystal structures, XRD is crucial for characterizing hydrates. It allows researchers to visualize the three-dimensional arrangement of atoms within a crystal lattice. The presence of water in a hydrate will affect the diffracted pattern, which can provide information on the type and amount of water present.
Each of these spectroscopic techniques contributes valuable data, leading to a well-rounded understanding of hydrate compositions. Yet, the complexity of hydrates means that often, no single method suffices. Instead, a combination of these techniques is recommended for thorough analysis and characterization.
Microscopic Techniques
Microscopic techniques further complement spectroscopic methods by providing visual insights into the morphology and surface properties of hydrates. These methods can help to scrutinize the physical characteristics and behavior of hydrates at micro and nanoscale levels.
- Scanning Electron Microscopy (SEM): SEM offers high-resolution imaging of hydrate microstructures. It can unveil surface features, such as pore sizes, shapes, and the arrangement of water molecules within the hydrateโs external morphology. Understanding these aspects can be critical in fields like material science, where the performance of hydrates is paramount.
- Transmission Electron Microscopy (TEM): Similar to SEM but capable of providing even higher magnification, TEM allows examination of the internal structure of hydrates. This technique can reveal the arrangement of water molecules and their interactions in real time, which can help address how external conditions affect hydrate stability.
- Atomic Force Microscopy (AFM): AFM provides topographical maps at an atomic level. By employing this method, researchers can observe the nano-scale properties of hydrates, which can be vital for applications that require precise interfacing between materials or when exploring the thermodynamic properties of hydrates.
The combination of microscopic examination alongside spectroscopic data cultivates a comprehensive understanding of hydrates, bridging the gap between theoretical predictions and real-world applications.
โThe fusion of spectroscopic and microscopic techniques provides a unique lens through which the complexity of hydrate systems reveals itself.โ
Challenges in Studying Hydrates
Studying hydrates presents many systematically intricate challenges that can significantly hinder research progress. The topic is essential for this article because it highlights the hurdles scientists face while investigating the complex nature of hydrate compounds. As we delve into this realm, understanding these challenges not only clarifies the existing limitations but also underscores the necessity for improved methods in hydrate research. This awareness can lead to more insightful findings and innovations in various fields, including environmental science, material engineering, and pharmaceuticals.
Experimental Difficulties
Experimental challenges in studying hydrates primarily arise from their inherent instability and sensitivity to environmental conditions. These hydrates often decompose upon exposure to even minor changes in temperature or pressure, making it difficult to maintain ideal studying conditions. For instance, when a hydrate loses its water content too rapidly, the resulting data can become unreliable. This instability paired with the usual data collection methods renders it crucial to develop better equipment and protocols.
Additionally, the solid-liquid phase transitions manifest complex behaviors that complicate experimental designs. The phase diagram can shift with varying experimental conditions, yet only so much can be accounted for in preliminary models. Researchers are therefore contending with a dynamic system. It thus becomes paramount for them to conduct trials in controlled environments to minimize stray variables. The use of expensive and sophisticated characterization tools can also escalate the costs associated with hydrate studies, presenting another barrier for researchers, particularly in resource-limited settings.
Some specific issues that researchers face include:
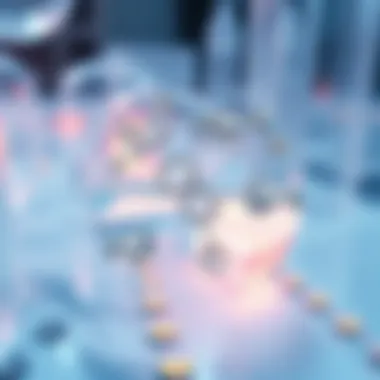
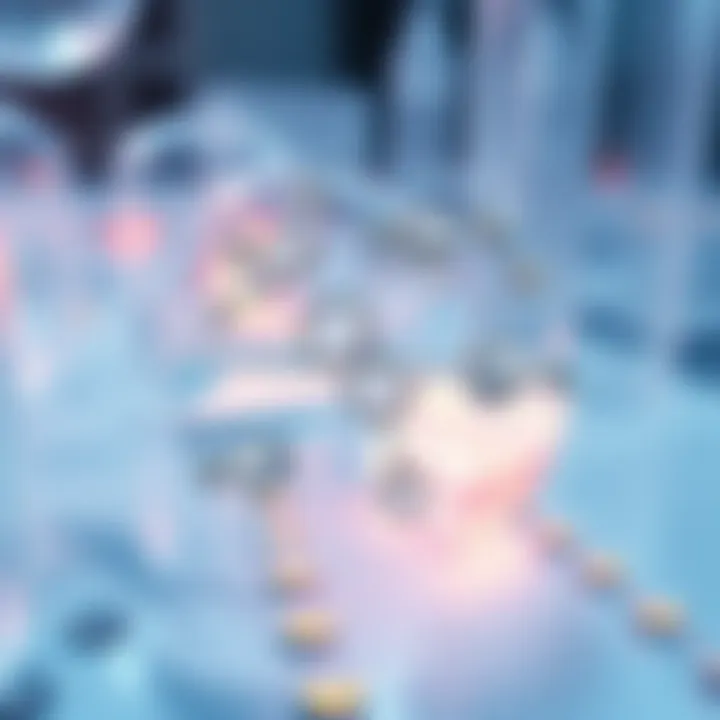
- Temperature Fluctuations: Maintaining consistent temperatures is vital, and variations can lead to either the formation or decomposition of hydrates.
- Pressure Changes: Changes in pressure can cause hydrates to release or absorb water, leading to misleading data.
- Sample Handling: Moisture-sensitive samples require special handling protocols; mishandling can lead to contamination or degradation.
Data Interpretation Issues
Interpreting data from hydrate studies can be fraught with complications as well. Due to the multifaceted nature of hydrates and their varied structures, arriving at conclusive results often feels like piecing together a scattered jigsaw puzzle. The presence of numerous chemical parameters affects hydrate stability, and this intricacy can cloud the interpretation process.
Furthermore, scientists are often working with limited data sets. For instance, the sensitivity of the hydrates can lead to incomplete information, making it hard to form comprehensive models or theories. Misinformation might inadvertently proliferate in findings, which disrupts the integrity of scientific knowledge.
A few specific challenges that hinder data interpretation include:
- Complex Phase Behavior: Hydrates can exhibit multiple phases, complicating the identification of stable forms.
- Sensitivity to Conditions: Data gathered from one experiment might not hold under different conditions, creating context-specific findings.
- Analytical Techniques Limitations: Many common methods used for analyzing hydrates might lack the resolution or specificity required to capture all relevant nuances.
โUnderstanding the limitations of our methods is the first step toward enhancing the future of hydrate research.โ
Current Research Trends in Hydrate Studies
The field of hydrate research has taken significant strides in recent years, reflecting the growing recognition of hydrates' potential across various disciplines. This section aims to illuminate the riveting landscape of current research trends within hydrate studies. Undoubtedly, the exploration of these topics will provide valuable insights for scholars, practitioners, and anyone keen to deepen their understanding of hydrates.
One area of exploration pertains to the advancements in synthesis techniques. Recent innovations have made it possible to produce hydrates with greater precision and efficiency. For instance, researchers are utilizing novel methods such as solvent-evaporation techniques and advanced crystallization processes which allow for enhanced control over the size and shape of hydrate crystals. This level of control is crucial for applications demanding specific properties, like in pharmaceuticals and energy storage systems. Improved synthesis not only elevates the quality of the materials but also significantly shortens the production time, boosting industrial applications.
Advancements in Synthesis Techniques
- Targeted Crystallization Methods: Techniques such as slow cooling or controlled evaporation have opened new doors for synthesizing hydrates with tailored properties. This is particularly important in fields like nanotechnology, where the size and uniformity of particles can dictate the material's overall performance.
- Use of Green Solvents: The trend toward environmentally friendly synthesis is also gaining momentum. Through the use of water or bio-based solvents, research indicates not only improved efficiency in the creation of hydrates but also reduced environmental impact.
"The innovations in synthesis techniques are not just a technical improvement; they signify a shift towards sustainable practices that can transform production processes.โ
- Automation in Synthesis: The introduction of automated systems has streamlined the synthesis of hydrates. This level of efficiency is crucial for scaling up laboratory findings to industrial levels, thus making it feasible to mass-produce hydrate-based materials.
By delving into innovative techniques, researchers can enhance the properties of hydrates to suit specific applications, which is pivotal in both scientific research and industrial usage.
Innovative Applications
Researchers are also exploring innovative applications of hydrates that extend beyond traditional uses. The versatility of hydrates is paving the way for fresh ideas, particularly in energy and environmental sectors.
- Energy Storage: Hydrates are being looked at for their capabilities in storing gases like methane. The ability to store energy effectively could play a crucial role in transitioning towards more sustainable energy solutions.
- Carbon Capture and Storage: Thereโs emerging evidence that hydrate formation might play a role in trapping carbon dioxide. This research could lead to breakthroughs in combating climate change by sequestering greenhouse gases effectively.
- Pharmaceutical Formulations: In the pharmaceutical realm, hydrates are making waves for their role in maintaining drug stability and controlling release rates. This iterative process is fundamental to effective drug delivery systems.
- Food Industry: Another fascinating area of research is the use of hydrates in the food industry. Hydrates can impact how ingredients interact, leading to improved texture and shelf-life for various products.
The exploration of these applications will greatly reshape our understanding and use of hydrate formulas, propelling the field of hydrate research in exciting new directions. As we look toward the future, one can't help but wonder what groundbreaking discoveries await us in this ever-evolving landscape.
Future Prospects of Hydrate Research
Hydrate research is at a crossroads, where traditional knowledge meets cutting-edge innovation. The anticipation surrounding this field isnโt just about uncovering new scientific truths; itโs also about the tangible benefits that could follow such discoveries. As industries scramble to find sustainable solutions to energy, environmental, and material challenges, the study of hydrates promises a wealth of opportunities.
One critical element of future hydrate research lies in its ability to contribute to energy solutions. Methane hydrate, in particular, has gained attention for its potential as a clean energy source. With ample reserves found in ocean sediments and permafrost, tapping into this resource could significantly affect the global energy landscape.
"While the extraction of methane from hydrates remains a technical challenge, ongoing research is striving to turn these potential resources into a real energy opportunity."
Potential Developments
Several key areas are poised for breakthroughs in hydrate research. First off, advancements in extraction technologies could lead to safer and more efficient methods for retrieving gas hydrates. This means moving from theoretical possibilities to practical applications that can actually be implemented at scale.
- Advanced Techniques: New drilling technologies are being developed that can minimize environmental disruption and increase recovery efficiency.
- Hybrid Solutions: Researchers are exploring methods that combine traditional extraction with renewable energy technologies. Imagine a scenario where offshore wind farms could power the extraction of methane from hydrates in a sustainable manner.
- Biological Methods: Thereโs an emerging interest in biological processes that can help in the stabilization and extraction of hydrates, reducing the energy costs associated with traditional extraction methods.
Given these developments, the future may hold innovative ways to harness the natural reservoirs of hydrates for both energy and material needs, transforming the way we think about energy sustainability.
Impact on Various Fields
The implications of continued hydrate research extend well beyond energy. Different sectors stand to feel the positive effects. In materials science, hydrates are being eyed for their unique properties. They can behave differently under various conditions, which makes them intriguing for developing new materials.
Some notable impacts include:
- Construction: Using hydrate compounds could result in more durable building materials, potentially reducing the carbon footprint of construction.
- Pharmaceuticals: Hydrates can be critical in drug formulation processes. Ongoing research into how hydrates affect drug solubility may lead to advancements in pharmaceutical development.
- Climate Science: Understanding how hydrates impact global warming is crucial. By studying their stability and role in carbon sequestration, we can gain insights into climate mitigation strategies.
As the landscape of hydrate research unfolds, itโs clear that the potential developments in this field have far-reaching consequences. From energy revolution to material improvements, the ongoing inquiry into hydrates stands to not only enrich scientific understanding but also to offer practical solutions to many of today's pressing challenges.
Ending
The conclusion of this article is pivotal as it encapsulates the essence of what has been explored throughout the discussion of hydrate formulas. From their intricate chemical compositions to their broad applications across scientific fields, this summation ties together all the threads of knowledge covered, ensuring that readers leave with a clear understanding of the subject's importance.
One of the central points of consideration is how hydrates affect various aspects of material science, biology, and environmental science. This article underscores the idea that hydrates are not just mere compounds brushed off in introductory classes but play a crucial role in multiple domains.
Summary of Key Insights
- Chemical Significance: Hydrates are characterized by their water molecules incorporated into a solid crystal. This inclusion greatly influences their chemical behavior, making them essential in various reactions and processes.
- Biological Relevance: The hydration processes in biological systems are vital. They regulate metabolism and influence cellular functions, emphasizing the need for a solid understanding of how hydrates operate within living organisms.
- Environmental Impact: Hydrates like methane hydrates play a critical role in understanding climate change and serve as potential energy resources. Their study is indispensable for developing strategies to address environmental challenges.
- Material Properties: In engineering, the thermal and mechanical properties of hydrates have far-reaching implications for material design. Understanding hydrate behavior can lead to innovative solutions in developing new materials.
- Research Trends: The landscape of hydrate research is evolving with advancements in synthesis and characterization techniques. This continuous evolution suggests that hydrates could provide solutions to emerging scientific problems.
Final Thoughts on Hydrates
In closing, the study of hydrates remains a dynamic and essential field. As we peel back more layers of their complexities, we unveil enormous potential that could influence future research and applications. It is clear that hydrates carry significant implications not only for chemistry but also for environmental science, engineering, and health. A detailed grasp of their properties and behaviors can open new doors in scientific advancements and innovations. This journey into hydrates is far from being merely academic; it holds real-world relevance that can shape how we approach contemporary challenges.
"Knowledge of hydrates provides insights that bridge various scientific disciplines and can lead to groundbreaking solutions, making them a subject worthy of ongoing focus and exploration."
The outlined insights and reflections on hydrates leave readers with an understanding that goes beyond textbooks, fostering a deeper appreciation for these compounds that intertwine with various aspects of life and technology. With ongoing research and development, the future looks promising for the science of hydrates.