Understanding Carbon Engineering and Capture Technologies
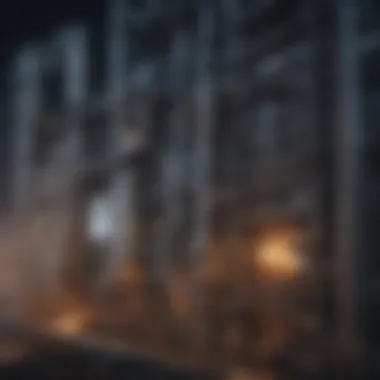
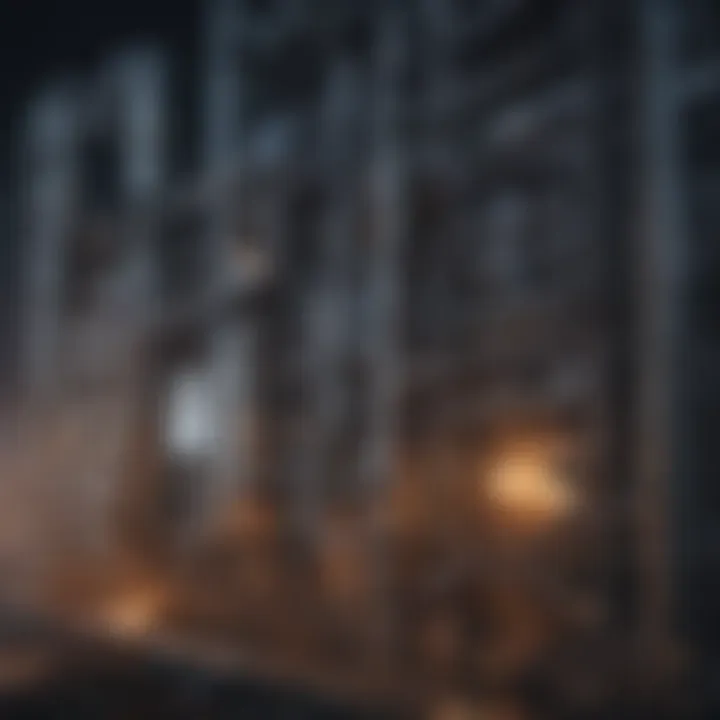
Intro
As global temperatures rise and the impacts of climate change become more pronounced, the need for effective strategies to reduce greenhouse gas emissions has become critical. Carbon engineering, specifically carbon capture technologies, offers a potential solution by capturing carbon dioxide before it is released into the atmosphere, or even directly from the air. This introductory section briefly sets the stage for a deeper exploration of how these technologies function and their role in sustainability.
In this discourse, we will dissect the fundamental principles behind carbon capture and how they align with broader environmental objectives. Understanding the mechanics of these technologies is essential for policymakers, researchers, and industry leaders focused on combating climate change.
Research Context
Background Information
Carbon capture technologies have evolved significantly over the last few decades. Their core principle revolves around the removal of carbon dioxide emissions produced from industrial processes, power generation, and atmospheric sources. Various methods, such as pre-combustion capture, post-combustion capture, and direct air capture, exhibit distinct advantages and limitations depending on the context of their application.
The study of these technologies has gained momentum partly due to international agreements aimed at reducing carbon footprints. Leading figures in environmental science have increasingly emphasized the necessity for innovative approaches to sequester CO2 effectively.
Importance of the Study
Understanding carbon engineering and capture technologies is crucial for multiple reasons:
- Mitigating Climate Change: As countries strive to meet their carbon reduction targets, these technologies offer a pathway to achieve these goals more efficiently.
- Economic Considerations: Effective carbon capture can also yield economic benefits, such as enhanced oil recovery and the generation of carbon-neutral fuels.
- Promoting Sustainable Practices: By integrating carbon capture into existing industrial processes, companies can reduce their environmental impact while maintaining profitability.
"The time to act on carbon capture is now, as it presents one of the few immediate options we have available."
Discussion
Interpretation of Results
Recent studies reveal that deploying carbon capture technologies at scale can lead to a substantial decrease in greenhouse gas emissions. Research indicates that the use of post-combustion capture technologies can reduce CO2 emissions from power plants by over 90%. Furthermore, current advancements, such as machine learning in optimizing capture processes, have shown promise in increasing efficiency and cost-effectiveness.
Comparison with Previous Research
Historically, the implementation of carbon capture was met with skepticism due to high operational costs and technological challenges. However, recent breakthroughs have revitalized interest in these methodologies.
Comparative studies highlight significant improvements between earlier models and state-of-the-art technologies. For instance, earlier carbon capture methods often faced difficulties in energy consumption, while newer designs focus on minimizing this factor, making them more viable for widespread application.
In summation, this exploration reveals that carbon engineering and carbon capture technologies play a foundational role in the global response to climate change. Their continual development and integration could define our capability to manage carbon emissions effectively in the future.
Prolusion to Carbon Engineering
Carbon engineering encompasses a set of technologies and methodologies aimed at reducing carbon emissions and promoting sustainability. This segment of the article focuses on the fundamental principles surrounding carbon engineering and why it deserves attention in the context of climate change mitigation. Given the pressing need for effective solutions to combat the rising levels of carbon dioxide in the atmosphere, carbon engineering serves as a critical component of a comprehensive strategy.
In discussing carbon engineering, the interaction between human activities and environmental impacts comes to the forefront. Various industrial activities, energy consumption, and transportation systems contribute significantly to greenhouse gas emissions. Addressing these contributions through carbon engineering not only aids in reducing harmful outputs but also aligns with global sustainability goals.
Definition of Carbon Engineering
Carbon engineering can be defined as the study and application of processes designed to manage and reduce carbon emissions from various sources. This field involves techniques that transform carbon dioxide into usable products or safely remove it from the atmosphere. The methods employed range from capturing carbon dioxide during industrial processes to enhancing the natural carbon sinks, such as forests and oceans.
More precisely, carbon engineering focuses on the development and implementation of technologies that enable carbon capture, utilization, and storage, commonly referred to as CCUS. By utilizing advanced materials, processes, and research, this field aims to facilitate sustainable solutions for carbon emissions reduction.
Importance in Climate Change Mitigation
The importance of carbon engineering in mitigating climate change cannot be overstated. Climate change represents an existential threat to ecosystems, human health, and socio-economic stability. Carbon dioxide and other greenhouse gases trap heat in the atmosphere, leading to global warming. Therefore, innovative approaches in carbon engineering provide a dual benefit. They directly address the issue of carbon emissions and contribute to the advancement of sustainable energy production.
Implementing carbon engineering practices has several notable advantages:
- Reduction of Atmospheric CO2 Levels: Efficient carbon capture technologies can significantly lower the amount of CO2 released from power plants and industrial facilities.
- Promotion of Circular Economy: By converting captured carbon into useful products, carbon engineering supports a circular economy model, reducing reliance on fossil fuels.
- Integration with Renewable Energy: Carbon engineering aligns with the broader movement towards renewable energy sources, enhancing the overall efficiency of energy systems.
"Innovative carbon engineering strategies transform environmental challenges into tangible solutions for sustainability."
In summary, the practice of carbon engineering is essential in the fight against climate change. By creating frameworks that facilitate carbon footprint reduction and increase the sustainability of energy systems, we move towards a future where development and environmental preservation coexist.
Understanding Carbon Capture
Carbon capture is an essential component in addressing the growing concerns of climate change. It aims to reduce the amount of carbon dioxide emitted into the atmosphere, thereby mitigating the greenhouse effect. Understanding how carbon capture works allows professionals and researchers to develop effective strategies to manage carbon emissions in various industrial applications. This process not only directly contributes to climate change mitigation but also supports global targets for reducing greenhouse gas emissions.
Mechanism of Carbon Capture
The mechanism of carbon capture involves several steps designed to efficiently separate CO2 from other gases produced during industrial processes. Typically, carbon capture systems capture CO2 from flue gases before it is released into the atmosphere. This is done through physical or chemical methods.
In general, there are three main stages in the process: capturing carbon dioxide, compressing it, and finally storing it. These stages ensure that CO2 is effectively isolated from other emissions, minimizing its environmental impact.
Types of Carbon Capture Technologies
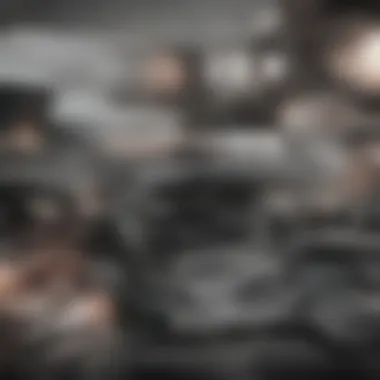
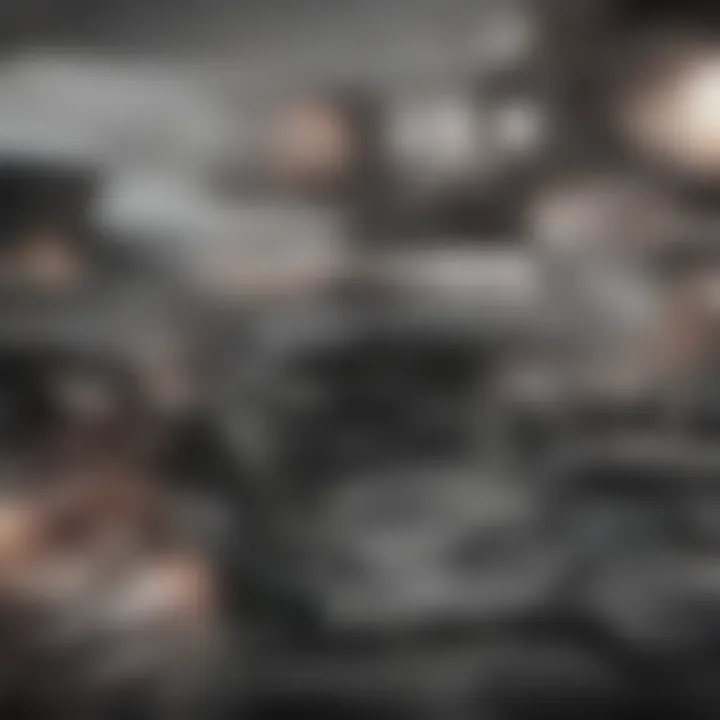
Carbon capture technologies can be categorized into several types, each with its own specific focus and application.
Pre-combustion Capture
Pre-combustion capture refers to a method that captures carbon dioxide before fossil fuels are burned. In this process, fuels are converted into a mixture of hydrogen and carbon dioxide. The carbon dioxide is then separated before combustion occurs.
A key characteristic of pre-combustion capture is its ability to produce hydrogen, which can be a cleaner energy source. This method is especially beneficial in integrated gasification combined cycle plants. However, pre-combustion capture requires significant energy input and investment in technology, which can be a downside in some scenarios.
Post-combustion Capture
Post-combustion capture focuses on capturing carbon dioxide after fossil fuels have been combusted. The CO2 is commonly removed from flue gas using solvents or absorbents that chemically bind with the gas.
The advantage of post-combustion capture is that it can be applied to existing facilities, making it a popular choice for retrofitting coal and gas-fired power plants. Its main disadvantage is that it may require considerable energy to separate CO2 from other combustion gases, which could reduce overall efficiency.
Oxy-fuel Combustion
Oxy-fuel combustion is a process that burns fossil fuels in an atmosphere of pure oxygen, rather than air. This leads to flue gases that consist mainly of water vapor and carbon dioxide, making it easier to capture the CO2.
The key characteristic of oxy-fuel combustion is the increased concentration of CO2 in the emissions, which facilitates capture. Additionally, it can be integrated into existing power plants with adjustments. However, the main disadvantage is the cost of producing pure oxygen, which can limit the scalability of this technology.
"The effectiveness of carbon capture technologies can significantly impact the strategies employed to mitigate climate change."
Understanding these various types of carbon capture technologies allows industries to choose the most effective methods for their specific needs. By investing in these technologies, organizations can enhance their sustainability efforts while contributing to global climate goals.
Techniques for Carbon Capture
Techniques for carbon capture play a vital role in mitigating climate change. They are significant because they help in reducing the amount of carbon dioxide released into the atmosphere. Carbon capture methods can be applied at various stages of industrial processes, making them versatile tools in the fight against global warming.
The advantages of these techniques include a direct impact on emissions reduction, potential economic benefits through carbon credits, and supporting compliance with environmental regulations. However, considerations such as efficiency, cost factors, and technological maturity must also be acknowledged.
Absorption Techniques
Absorption techniques involve dissolving carbon dioxide in a liquid solution. The most common solvent used is an amine solution, which reacts with carbon dioxide to form a compound, effectively removing it from the gas stream. This process is generally carried out in large equipment known as scrubbers.
Notably, absorption techniques are widely used in industrial settings, particularly in power plants and chemical manufacturing. They are well-established and can be scaled to meet industrial requirements.
One challenge of absorption is the energy required to regenerate the solvent after it becomes saturated with carbon dioxide. While effective, this energy consumption can influence the overall efficiency and cost of the carbon capture process.
Adsorption Techniques
Adsorption relies on solid materials to capture carbon dioxide from the gas phase. In this method, CO2 molecules adhere to the surface of solid adsorbents, such as activated carbon or zeolites. The process can be conducted at ambient temperatures, making it energy efficient compared to other methods.
The key benefits of adsorption include the ability to selectively capture CO2 and the potential for lower operational costs. Furthermore, advancements in material science continue to enhance the performance of various adsorbents. However, the main challenge lies in the potential limitations of adsorbent capacity and the regeneration process, which can complicate implementation.
Membrane Separation Techniques
Membrane separation techniques utilize specially designed membranes that allow for the selective passage of carbon dioxide while blocking other gases. This process can operate continuously, making it suitable for various industrial applications. Membranes can be made from various materials, including polymeric and ceramic compounds.
These techniques offer significant advantages, including lower energy requirements and a smaller footprint compared to traditional methods. However, the performance of membranes can be affected by contaminants in the gas stream, necessitating pre-treatment steps in some cases.
The development of new membrane materials continues to advance the efficiency and effectiveness of this method for carbon capture.
Chemical Looping
Chemical looping is a relatively novel approach to carbon capture where a solid oxygen carrier reacts with fuel to produce energy and carbon dioxide in a separate chamber. The main advantage of this technique is that it can inherently separate carbon dioxide from other gases without the need for additional processing.
By using chemical looping, industries can achieve high carbon capture efficiency while maintaining lower energy consumption. However, this technology is still under research and may face scalability challenges. More studies are required to assess its feasibility for widespread use in various applications.
In summary, the techniques for carbon capture are diverse and critical in addressing the pressing issue of climate change. Each method has its strengths and weaknesses that must be carefully assessed to determine the most appropriate applications in different sectors.
Applications of Carbon Capture
Carbon capture technologies play a vital role in addressing the pressing issue of climate change. They have numerous applications that span several industries and sectors. By effectively removing carbon dioxide from atmospheric emissions, these technologies not only reduce greenhouse gas levels but also promote sustainability. The integration of carbon capture in various applications presents both benefits and considerations that are crucial to the ongoing conversation about energy and environmental policy.
Industrial Applications
In the industrial sector, carbon capture is essential in mitigating emissions from processes such as cement production, steel manufacturing, and chemical processing. These industries are typically significant contributors to CO2 emissions due to their high energy consumption and reliance on fossil fuels. Implementation of carbon capture systems in these industries can lead to the following benefits:
- Emission Reduction: By capturing carbon dioxide before it releases into the atmosphere, these technologies significantly lower the overall carbon footprint of industrial plants.
- Compliance and Reputation: Adhering to increasingly stringent regulations on emissions enhances corporate responsibility and can boost a companyβs public image.
- Resource Recovery: Captured CO2 can be repurposed for other industrial processes, such as enhanced oil recovery or the production of synthetic fuels. This not only reduces waste but also adds economic value.
Energy Generation
The energy generation sector is another crucial area where carbon capture technologies can make a substantial impact. Power plants, especially those that use coal or natural gas, are major sources of carbon dioxide emissions. Incorporating carbon capture here allows for:
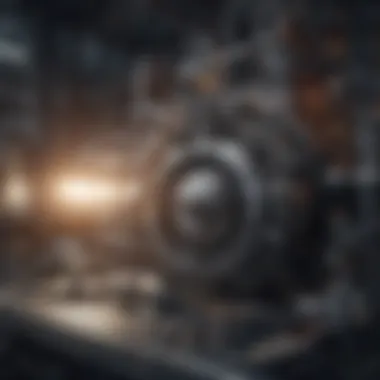
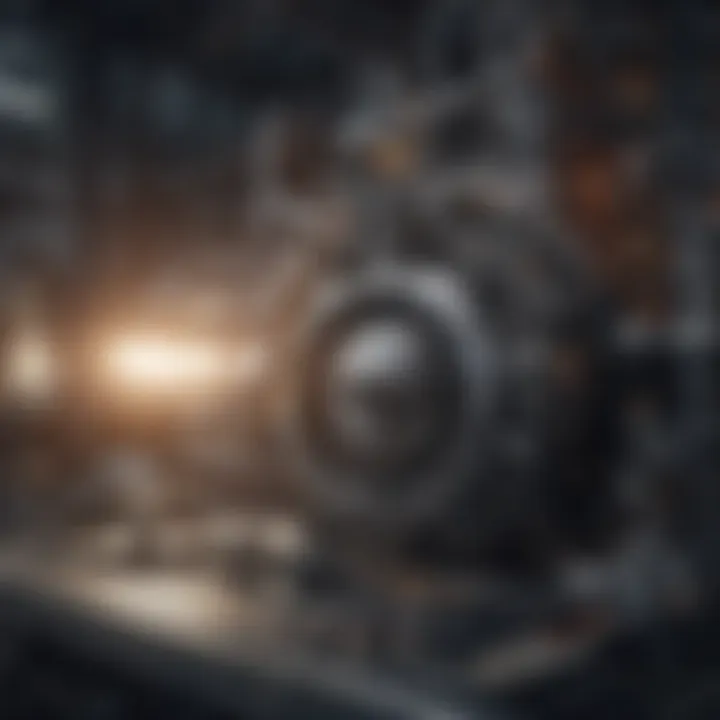
- Clean Energy Transition: Capturing emissions from traditional fossil fuel power generation can help facilitate a gradual shift towards cleaner energy sources without abruptly shutting down existing infrastructure.
- Carbon Neutrality: By employing carbon capture at power plants, operators can make strides toward achieving net-zero emissions, crucial for international climate goals.
- Innovative Solutions: New methods, including carbon capture from bioenergy, can further enhance the sustainability of energy generation, effectively creating a cycle of carbon reuse.
Environmental Remediation
Beyond industrial and energy sectors, carbon capture technologies have noteworthy applications in environmental remediation. The ability to sequester carbon dioxide plays a significant role in:
- Restoration of Ecosystems: CO2 capture can be coupled with bioremediation efforts to restore natural environments, aiding in the recovery of ecosystems that have suffered due to industrial pollution.
- Carbon Sequestration Projects: Implementing capture technologies in geological formations can help mitigate atmospheric carbon levels, contributing to long-term sustainability initiatives.
- Public Health: Reduced atmospheric CO2 correlates with improved air quality, leading to better health outcomes for communities near industrial sites.
"Carbon capture technologies can fundamentally change how we approach both industry and environmental stewardship. Their applications are both numerous and essential toward achieving sustainability goals."
In summary, the applications of carbon capture technologies are diverse and strategically important in combating climate change. By effectively using these technologies across various sectors, it is possible to achieve significant reductions in carbon emissions. Industry practitioners and policymakers must focus on integrating these solutions into their operations to pave the way for a sustainable future.
Role of Carbon Engineering in Sustainability
Understanding the role of carbon engineering in sustainability is essential for comprehending how we may confront the challenges of climate change. Carbon engineering refers to the suite of technologies and practices designed to capture, utilize, and store carbon dioxide emissions. In this context, carbon engineering is significant for several reasons. Firstly, it provides a pathway to reduce greenhouse gas emissions, which is crucial for meeting climate targets. The successful integration of carbon capture technologies can play a pivotal role in achieving carbon neutrality by addressing emissions from hard-to-abate sectors.
Integration with Renewable Energy Sources
Integrating carbon capture with renewable energy sources is a vital aspect of enhancing sustainability. Renewable energy, such as solar and wind, produces low to zero carbon emissions. However, their intermittency poses challenges for grid stability. By capturing carbon from energy generation activities, it is possible to create synergies that improve efficiency. For example, biogas from organic waste can be integrated into renewable gas systems, providing cleaner energy and further reducing carbon emissions.
Here are some key considerations in this integration:
- Utilization of surplus energy: During periods of low energy demand, surplus energy from renewables can be used for carbon capture processes, effectively storing energy in chemical form while reducing emissions.
- Hybrid systems: By combining fossil fuel generation with renewable sources, a hybrid system can optimize the carbon capture process while minimizing emissions. This strategic blend can bolster grid reliability and enhance energy security.
- Innovative storage solutions: Renewable energy sources can be harnessed to power the carbon capture process itself, making it even more sustainable. This includes using wind or solar energy to operate carbon capture units, further lowering the carbon footprint of energy generation.
Enhancing Energy Efficiency
Carbon engineering also enhances energy efficiency within existing systems. High energy consumption often correlates with high carbon emissions. By implementing carbon capture technologies, energy plants can operate more effectively. These technologies can directly lower the energy required for processes, creating a positive feedback loop of efficiency and emissions reduction.
Consider the following benefits of increased energy efficiency through carbon capture:
- Process optimization: Carbon capture technologies can lead to more efficient combustion processes, resulting in lower fuel consumption and reduced operational costs.
- Waste heat recovery: Advanced carbon capture systems make use of waste heat from industrial processes, converting it into usable energy. This not only minimizes emissions but also makes the overall system more efficient.
- Industrial retrofitting: Existing energy plants can be retrofitted with carbon capture technologies, minimizing the need for new infrastructure while enhancing efficiency and reducing emissions.
"Carbon engineering represents not only a technological solution but also an economic opportunity, fostering sustainable practices that encourage innovative thinking and collaboration across sectors."
Overall, the role of carbon engineering in sustainability is multifaceted, emphasizing the importance of integrating renewable energy sources and enhancing energy efficiency. The application of these technologies can lead to significant reductions in carbon emissions, making a substantial contribution to global climate change mitigation efforts.
Technological Innovations in Carbon Capture
Technological innovations in carbon capture are crucial for enhancing the effectiveness and efficiency of carbon capture methods. These advancements help in reducing greenhouse gas emissions and fighting climate change. Innovations focus on increasing the capture rate, lowering the costs associated with carbon capture systems, and improving the overall integration within existing industrial processes. This section will explore two key areas of innovation: advancements in material science and emerging capture technologies.
Advancements in Material Science
Material science plays a pivotal role in the development of more efficient carbon capture technologies. New materials are continually being researched and developed to improve the efficiency of carbon capture systems.
- New Sorbents: The introduction of advanced sorbent materials has enhanced the absorption of carbon dioxide from flue gases. These materials tend to have higher selectivity and can capture more CO2 under various operating conditions. Examples include metal-organic frameworks (MOFs) and zeolites, which are showing significant promise due to their porous structure.
- Nanotechnology: The integration of nanotechnology has led to the creation of catalysts that are more effective in carbon capture processes. These catalysts can operate at lower energy requirements, thereby making the overall process more sustainable.
- Cost-Effective Materials: Researchers are aiming to find materials that not only perform well but are also economically viable. Natural materials or recycled inputs are being considered to create cost-effective alternative sorbents, reducing the burden of high operational costs.
Overall, advancements in material science are vital. They make carbon capture technologies more practical and aligned with sustainability goals.
Emerging Capture Technologies
Emerging technologies in carbon capture are critical for addressing the global challenge of reducing CO2 emissions. Several innovative technologies are at various stages of research and development, each having unique methodological approaches to capturing carbon.
- Direct Air Capture (DAC): This technology extracts carbon dioxide directly from the atmosphere. It is especially significant as it addresses existing greenhouse gas levels rather than just future emissions. By using large fans and chemical processes, DAC can provide a viable method for climate mitigation.
- Bioenergy with Carbon Capture and Storage (BECCS): This process integrates biomass energy generation with carbon capture. It captures CO2 emitted during the energy generation process, allowing for negative emissions when the biomass feedstock is grown sustainably.
- Carbon Capture Utilization (CCU): This approach focuses on transforming captured CO2 into useful products. Applications can range from creating synthetic fuels to producing materials like concrete, which can further sequester the carbon.
These technologies highlight the potential pathways to enhance carbon capture capabilities significantly. Innovations must continue to evolve as the necessity for effective climate strategies grows.
"Technological advancements are the backbone of developing effective carbon capture solutions that can be integrated seamlessly into our current industrial framework."
With ongoing research and development, the future of carbon capture technologies holds promise for significant improvements in mitigating climate impacts.
Challenges in Carbon Capture Implementation
The implementation of carbon capture technologies presents significant hurdles that require careful consideration. This section will delve into the various challenges faced in the deployment of carbon capture methods. Understanding these challenges is essential as they influence the feasibility and efficiency of capturing carbon emissions across industries.
Economic Viability
One of the main barriers to widespread adoption is economic viability. The costs associated with carbon capture can be substantial. These encompass initial setup costs, operational expenses, and maintenance of capture systems. According to recent studies, the price of capturing one tonne of carbon dioxide can range widely, depending on the method employed and scale of the operation.
Several factors impact this economic landscape. First, the price of carbon credits influences investment decisions. When carbon pricing is low, the incentive to incorporate capture technologies diminishes. Additionally, the technology needs to advance to lower costs without compromising efficiency. A
"A balance between costs and effectiveness is crucial for determining both short-term and long-term success in capturing carbon emissions."
Further, securing financing for these technologies can be a challenge, especially in regions where public and private funds are limited. Companies and governments may hesitate to make long-term investments without guarantees of prices for carbon capture becoming competitive.
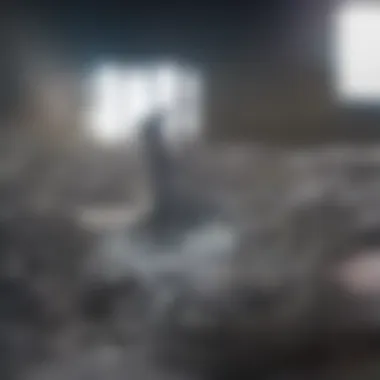
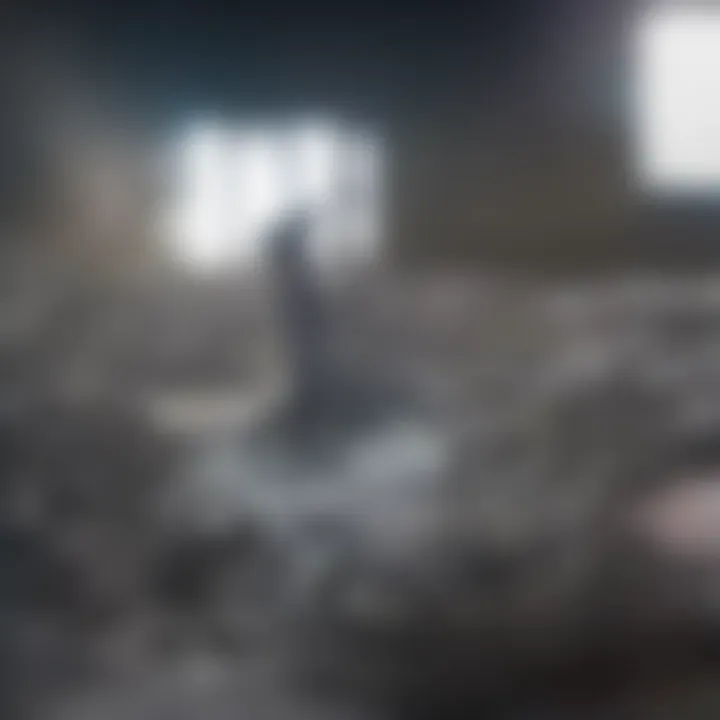
Regulatory and Policy Framework
The regulatory environment plays a critical role in carbon capture practices. Effective policies and frameworks can bolster the development and implementation of these technologies. However, inconsistent regulations can hinder progress, creating uncertainty for potential adopters.
Lack of standardized guidelines makes it difficult for businesses to navigate compliance. Regulations vary significantly by region, impacting the viability of employing carbon capture strategies. For some companies, navigating complex permits and compliance measures requires considerable time and resources.
Moreover, government support, through incentives or grants, can drive development. The absence of such support may stall projects that could otherwise lead to substantial carbon reductions. Industry stakeholders are advocating for clearer frameworks that foster wider adoption and improve project clarity.
Technological Limitations
Technological limitations represent another significant barrier in capturing carbon. The existing technologies are effective under specific conditions but may not be universally applicable. Different industries produce carbon emissions in various forms, which can affect the capturing process efficiency.
For instance, some capture methods may struggle with low concentrations of carbon dioxide found in certain emissions. This necessitates continued research and development to innovate new methods and materials that can overcome current limitations.
Additionally, scalability remains a concern. Some techniques that work effectively in pilot plants encounter issues when applied on a larger scale. The integration of these technological solutions into existing infrastructures can also pose challenges.
In summary, while carbon capture technologies are promising tools in the fight against climate change, various challenges must be addressed. Economic viability, regulatory frameworks, and technological limits are key issues needing attention for successful implementation and broader acceptance.
Future Prospects of Carbon Engineering
The future of carbon engineering and carbon capture technologies is of significant interest, especially as the global community grapples with climate change impacts. The urgency to reduce greenhouse gas emissions makes this field crucial. Understanding future prospects allows for more informed decision-making by stakeholders in policy, technology, and industry.
Scaling Up Carbon Capture Technologies
Scaling up carbon capture technologies is essential for reaching global climate goals. Technologies developed in the lab must transition to full-scale applications. This involves overcoming various challenges such as cost, efficiency, and infrastructure requirements.
Some key points about this scaling include:
- Investment Requirements: Significant financial resources are necessary. Public and private sectors must collaborate to fund large-scale projects.
- Efficiency Improvements: Current technologies need to be optimized for higher capture rates. Researchers are focusing on improving efficiencies in various methods. Assumption of performance at scale is different, hence continuous testing is vital.
- Infrastructure Developments: An integral part of scaling is the infrastructure to transport and store captured CO2. In many regions, insufficient infrastructure exists, which hinders progress. Well-planned logistics are necessary for effective implementation.
By addressing these areas, the potential for widespread adoption of carbon capture technologies increases. Such advancements can facilitate the reduction in atmospheric CO2 levels, contributing to climate mitigation efforts.
Global Cooperation and Research Initiatives
Global cooperation and research initiatives are vital in advancing carbon engineering technologies. International collaboration fosters knowledge exchange and resource sharing. Various nations can pool expertise and resources to address this global challenge effectively.
Considerations around cooperation include:
- Shared Goals: Nations must align on climate objectives. The Paris Agreement is an example of a platform where countries commit to emission reductions collectively.
- Joint Research Programs: Initiatives like the Carbon Capture and Storage Research Fund enable joint studies. Collaborative research not only enhances technology but also reduces duplicative efforts globally.
- Knowledge Sharing: Forums such as the Global CCS Institute promote sharing innovative practices. Lessons learned from one region can inform efforts in another, accelerating technological advancements.
Case Studies in Carbon Capture
Case studies play a critical role in the advancement of carbon capture technologies. They provide real-world examples of implementation, offering insights into the successes and challenges faced in various contexts. By analyzing these cases, professionals and researchers can better understand the practical applications, limitations, and potential improvements necessary for widespread adoption. The study of these cases illuminates the ways carbon capture can be effectively integrated into existing systems, which is vital for climate change mitigation efforts.
Successful Implementations
Successful implementations of carbon capture technologies highlight the promise of these systems in various sectors. Several notable projects demonstrate effective strategies and outcomes:
- Sleipner CO2 Storage Project: This project in Norway captures CO2 from natural gas processing and stores it under the North Sea. Since its start in 1996, it has successfully sequestered over 1 million tons of CO2 annually, showcasing long-term storage capabilities.
- Boundary Dam: Located in Canada, this coal-fired power plant captures around 90% of its CO2 emissions. The captured CO2 is repurposed for enhanced oil recovery, thus providing both a reduction in emissions and an economic benefit.
- Petra Nova: An initiative in Texas that captures over 1.6 million tons of CO2 each year from a coal power plant. The project has gained attention for its innovative approach to integrating carbon capture with oil recovery, illustrating how carbon capture can be economically viable.
These implementations serve not just as evidence of technical feasibility, but also as models for future projects. They provide valuable lessons on optimizing processes, managing costs, and addressing community concerns.
Lessons Learned from Failures
Failures in carbon capture projects are equally instructive, revealing what does not work and why. Understanding these missteps is crucial for advancing technology and policy. Some key lessons include:
- Technical Challenges: Issues such as equipment failures or insufficient capture rates can arise. For instance, the Cement Supervisory Carbon Capture Project faced significant setbacks due to underperformance in capturing carbon dioxide, highlighting the necessity for robust testing and deployment phases.
- Economic Feasibility: High operational costs can deter implementation. Many projects saw financial difficulties due to fluctuating market prices for captured carbon. Evaluating the economic landscape prior to implementation can prevent future pitfalls.
- Regulatory Hurdles: Compliance with stringent regulations can impede progress. Projects have stalled or been canceled because of evolving government policies and environmental standards. Continuous dialogue with regulatory bodies is essential for navigating these challenges.
In summary, both successful and failed case studies in carbon capture provide a comprehensive framework for understanding the complexity of these technologies. They highlight what works, what needs improvement, and how approaches can be refined for better outcomes in the future.
Culmination
The conclusion serves as an essential synthesis of the findings presented throughout this article. In assessing carbon engineering and carbon capture technologies, it is evident that these fields not only provide solutions to combat climate change but also facilitate the transition toward a more sustainable future.
Summary of Key Insights
This article highlighted several critical points regarding carbon engineering. It emphasized the techniques and technologies that successfully capture atmospheric carbon dioxide. The mechanism of carbon capture, whether through absorption or membrane separation, is pivotal in reducing greenhouse gas emissions. We discussed different applications ranging from industrial uses to energy generation and environmental remediation.
Moreover, we explored the challenges faced in implementing these technologies. These include economic viability and the regulatory framework necessary for adoption. Understanding these insights equips professionals in the environmental science community with the knowledge to advance the carbon capture agenda effectively.
Call to Action for Professionals and Researchers
Professionals and researchers are urged to undertake further studies in carbon capture methodologies. Collaborations among sectors could enhance innovations and deployment. Therefore, contributions to the dialogue on policy improvements are vital.
Research should focus on:
- Enhancing the efficiency of existing carbon capture technologies.
- Developing cost-effective solutions that can be applied in diverse industries.
- Sharing insights on the successes and failures of implemented projects to promote knowledge transfer.
Engaging with governmental agencies and private sectors can create an ecosystem that supports robust carbon management strategies. Those involved in engineering, environmental science, or related fields have a pivotal role in shaping a sustainable environment through carbon capture initiatives.