Exploring the Leaf Spectrometer: Principles and Applications
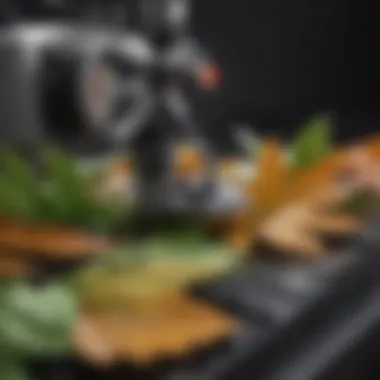
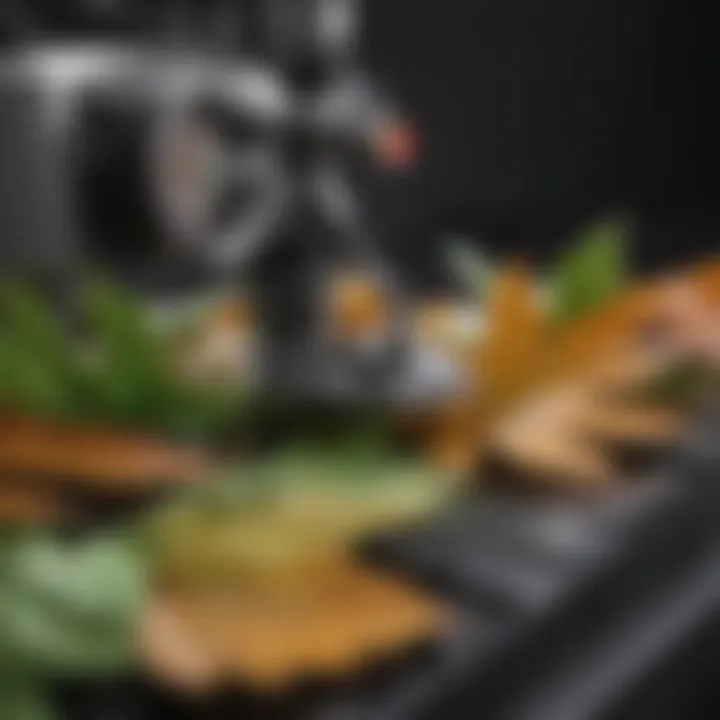
Intro
Leaf spectrometry stands as a fascinating field within plant research. It bridges the gap between optical physics and botany, revealing insights into plant health and ecosystem functioning. This article dives into the intricate workings of leaf spectrometers, unraveling their core principles, technical aspects, and diverse applications in scientific inquiries.
Research Context
Background Information
Leaf spectrometers are instrumental tools that measure the reflection and absorption of light by plant leaves. This measurement provides valuable information about chlorophyll content and other pigments, which are critical for photosynthesis. The basic principle involves shining light across various wavelengths onto a leaf and capturing the reflected light. Each pigment absorbs light at specific wavelengths, allowing researchers to determine the health of the plant and its ability to synthesize energy.
Importance of the Study
Understanding the principles behind leaf spectrometers is vital for multiple reasons. First, they offer a non-destructive method for analyzing plants. This is crucial as destructive methods can limit the longevity of a sample. Secondly, the detailed information these instruments provide can aid in agricultural productivity, conservation efforts, and ecological research. By comprehensively analyzing leaf pigments, scientists can assess phytochemical changes in response to environmental stressors and climate change.
Optical Principles of Leaf Spectrometry
Leaf spectrometers operate based on the interaction of light with plant pigments. The spectrum of light absorbed by chlorophyll is particularly significant.
- Chlorophyll a absorbs light primarily in the blue and red wavelengths.
- Chlorophyll b absorbs light in the blue and orange wavelengths.
The unique absorption spectra of these chlorophyll forms enable researchers to understand various physiological states of the plant.
"The absorption spectra of chlorophyll reflect the efficiency of photosynthesis and provide insights into plant health."
In addition to chlorophyll, other pigments such as carotenoids also play a vital role in photosynthesis and plant health assessment, especially during senescence or stress.
Technical Specifications
Modern leaf spectrometers come equipped with various features aimed at improving data quality. These include:
- High spectral resolution: Allows for detailed analyses, differentiating between pigments accurately.
- Portable designs: Facilitates field research without the need for a lab setup.
- User-friendly interfaces: Makes it easier for researchers of all backgrounds to utilize the technology effectively.
These advancements enhance the overall functionality and applicability of leaf spectrometers in diverse research settings.
Applications in Scientific Research
Leaf spectrometers have a wide range of applications:
- Agricultural Research: Monitoring crop health and determining optimal harvesting times.
- Environmental Science: Assessing the impact of pollutants on plant physiology.
- Ecological Studies: Understanding plant responses to climate variability and ecosystem changes.
By integrating the data obtained from leaf spectrometry with other research methodologies, scientists can develop strategies to address challenges such as food security and biodiversity loss.
Ending
This exploration of leaf spectrometers emphasizes their significance in plant research. Understanding their optical principles and technical specifications opens doors to numerous applications across various scientific domains. As technology continues to advance, the role of leaf spectrometry will undoubtedly expand, leading to even deeper insights into plant physiology and ecology.
Prolusion to Leaf Spectrometry
Leaf spectrometry plays a pivotal role in the field of plant sciences, serving as a sophisticated tool for analyzing the biochemical properties of plant leaves. This discipline utilizes the principles of spectroscopy to assess various elements, including chlorophyll content and other pigments that contribute to a plant's photosynthetic capacity. The importance of leaf spectrometry extends beyond mere academic inquiry; it facilitates practical applications in agriculture, environmental science, and ecological research, enhancing our understanding of plant health and ecosystem dynamics.
Modern techniques in leaf spectrometry offer significant benefits. They enable precise measurement of leaf constituents, thus providing valuable insights into the physiological states of plants. With advancements in technology, these methods have become more accessible, allowing researchers and practitioners to monitor plant health more effectively. Understanding the principles behind leaf spectrometry is crucial for applying it effectively in practical scenarios.
Historical Context
The roots of spectroscopy can be traced back to the 17th century, but leaf spectrometry as a distinct field has developed over the past few decades. Initial studies focused primarily on the reflection and absorption properties of leaves in relation to light wavelengths. The pioneering work by scientists such as Robert Hooke and later, more detailed studies in the 20th century, laid the groundwork for the analysis of plant materials using spectroscopic techniques. Developments in technology have been instrumental, leading to the introduction of portable spectrometers that make field analysis feasible.
Significance in Modern Science
In the contemporary scientific landscape, leaf spectrometry has emerged as a critical tool for several reasons. First, it allows for non-destructive analysis, meaning that plants can be studied without causing harm. This is particularly important in conservation efforts and agricultural practices where the health of the plant must be maintained. Moreover, the ability to analyze chlorophyll absorption spectra has significant implications for monitoring crop health and understanding stress responses in plants.
Furthermore, leaf spectrometry correlates directly with environmental factors, such as nutrient availability and climatic conditions. Researchers can utilize these correlations to assess the health of forests and agricultural fields, which are both critical for biodiversity and food security. As scientists strive to understand the growing challenges posed by climate change, the insights provided by leaf spectrometry will be indispensable for developing adaptive strategies.
Fundamental Principles of Spectroscopy
Understanding the fundamental principles of spectroscopy is essential for grasping how leaf spectrometers operate. Spectroscopy plays a crucial role in analyzing the light characteristics emitted or absorbed by different materials, including leaves. This foundational knowledge allows researchers to interpret the information derived from spectrometric data effectively.
The key elements of spectroscopy involve the study of light and its interaction with matter. When light interacts with a leaf, it can be absorbed, reflected, or transmitted, and these interactions are the basis for various spectroscopic techniques. By analyzing the light spectra, scientists can extract valuable insights regarding plant health, species differences, and environmental conditions.
Basic Concepts of Light and Spectra
Light is often described as a spectrum made up of various wavelengths. The visible spectrum is the range that the human eye can perceive, spanning roughly from 380 to 750 nanometers. Each wavelength correlates to a specific color, influencing how plants interact with the light.
Plants primarily use light for photosynthesis, wherein they absorb specific wavelengths, mainly in the blue and red regions of the spectrum. The relationship between light and plant physiology is foundational in leaf spectrometry. Color bands corresponding to different wavelengths can reveal much about chlorophyll content and overall plant health.
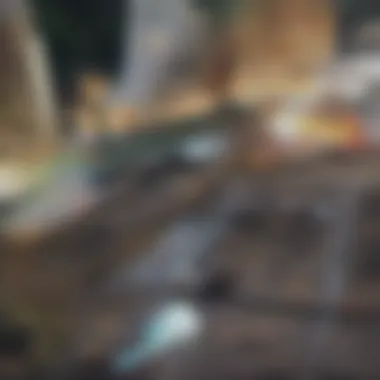
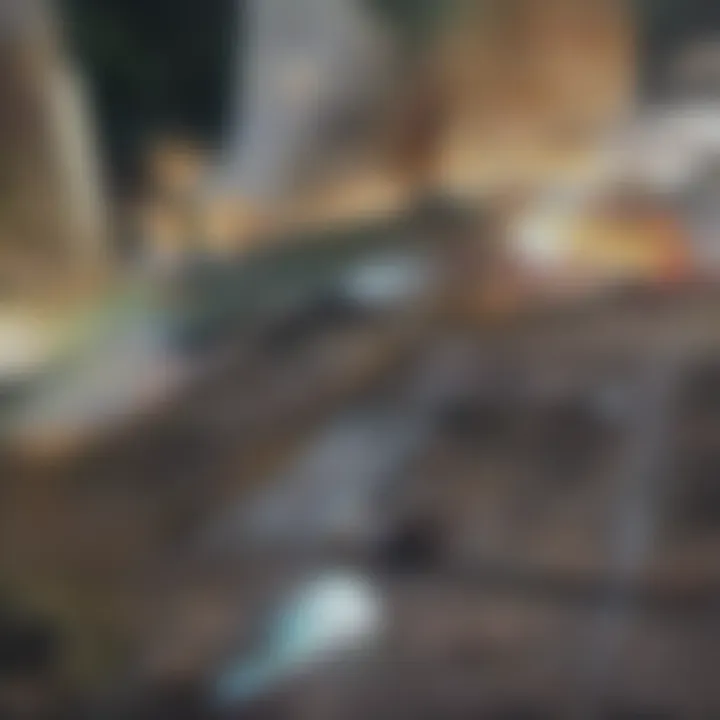
In spectroscopy, spectra can be classified into continuous and line spectra. A continuous spectrum shows all wavelengths within a range, while line spectra exhibit distinct and separated wavelengths. The principle of energy levels in atoms leads to these line emissions, as different leaves will emit or absorb light at distinct wavelengths, characteristic of their specific pigment composition.
"In the study of plant physiology, understanding light interaction is fundamental to interpreting spectroscopic data effectively."
Interaction Between Light and Leaf Material
When light strikes leaf material, several phenomena occur. Absorption is when certain wavelengths of light are taken up by the leaf, mainly due to pigments like chlorophyll. Reflectance involves light bouncing back off the leaf surface, while transmittance refers to light passing through the leaf tissue. Each of these interactions provides unique data points.
The composition of leaf material significantly influences these interactions. Factors such as leaf thickness, surface texture, and pigment concentration determine how light behaves when it encounters a leaf. For example, a leaf with high concentration of chlorophyll will absorb more light in the blue and red spectra compared to a leaf lacking in this pigment.
Generally, different leaf types exhibit varied absorption and reflectance patterns, making these measurements valuable for identifying species and assessing health. Spectrometers can capture this diverse information, converting it into data that researchers can analyze for trends and anomalies in plant health.
In summary, the principles of spectroscopy provide a robust framework for understanding plant interactions with light. By comprehending how light behaves with leaf material, researchers can unlock deeper insights into plant physiology and ecosystem dynamics.
Types of Leaf Spectrometers
Understanding the types of leaf spectrometers is crucial for comprehending how they are utilized in various scientific fields. Each type has its unique advantages and specifications, catering to different research needs. With the development of technology, these spectrometers play a significant role in analysesβbe it in the field or a controlled laboratory environment.
The two main categories are field spectrometers and laboratory spectrometers. \n This division is based on their design, use cases, and operational environments. Each type provides specific benefits but also comes with its own set of challenges. Knowing these distinctions can help researchers select the appropriate spectrometer for their studies.
Field Spectrometers
Field spectrometers are designed for in-situ measurement, allowing scientists to analyze leaves directly in their natural environment. This type of spectrometer is generally portable and designed to withstand varying environmental conditions.
Key advantages of field spectrometers include:
- Mobility: They can be easily transported, facilitating data collection in remote locations.
- Real-Time Analysis: Instant results can be crucial for understanding ecological dynamics.
- Cost-Efficiency: Field spectrometers are often less expensive than their lab counterparts.
However, they have limitations. Environmental factors like light scattering, humidity, and temperature can affect measurement accuracy. A common challenge is calibrating these devices adequately, often complicated in varying field conditions. Thus, researchers using field spectrometers must be adept at interpreting results within these limitations.
Laboratory Spectrometers
Laboratory spectrometers are intended for controlled settings, where conditions can be managed for more precise measurements. They often have superior resolution and can capture finer details in the leaf's spectral signature.
Benefits of laboratory spectrometers include:
- High Precision: Enhanced resolution leads to accurate and detailed spectral data.
- Controlled Conditions: Researchers can eliminate environmental variables that might skew results.
- Advanced Features: Many lab spectrometers come equipped with multiple detection techniques, improving data acquisition.
Despite their advantages, these spectrometers are stationary and less versatile compared to field spectrometers. Their higher cost and the need for specialized facilities can also restrict their use in some research contexts. For precise analyses based on controlled factors, however, laboratory spectrometers earn their value.
The choice between field and laboratory spectrometers is often governed by the specific objectives of the research study. Knowing these parameters helps in selecting the right equipment for effective leaf analysis.
By understanding the types of leaf spectrometers, researchers can align their tools with their objectives, optimizing their studies to garner meaningful results.
Technical Specifications
The technical specifications of a leaf spectrometer underscore its effectiveness in scientific research. These specifications provide researchers with crucial information about its capabilities and limitations. The chosen spectrometer's features directly influence the accuracy of measurements and the quality of data collected during experiments. Understanding these specifications allows users to make informed decisions tailored to their specific applications in plant analysis.
Wavelength Range and Resolution
The wavelength range is a key criterion in the performance of leaf spectrometers. It refers to the spectrum of light that the device can detect. For leaf analysis, a range from ultraviolet to near-infrared light is essential. Many chlorophyll absorption peaks occur in the visible range, typically from 400 to 700 nanometers, while additional information can be gathered in the near-infrared range, spanning from 700 to 1100 nanometers. A wider wavelength range enables comprehensive analysis of leaf properties, such as pigments and structural characteristics.
Resolution is equally important. It defines the ability of the spectrometer to distinguish between closely spaced wavelengths. High resolution allows for precise identification of absorption features related to specific compounds within the leaves. Inadequate resolution can lead to overlapping signals, thus complicating the interpretation of data. Thus, choosing a spectrometer with sufficient resolution is critical when analyzing leaf chemistry and physiology.
Calibration Techniques
Calibration is a vital process for ensuring the accuracy of measurements taken by leaf spectrometers. This involves standardizing the spectrometer's response against known reference materials. Without proper calibration, results can be misleading. Several calibration techniques exist, each serving various purposes.
- Dark Calibration: This method accounts for the background noise and determines the baseline response of the spectrometer. By collecting data without light, researchers can filter out extraneous signals.
- White Calibration: This involves capturing the spectrometerβs response using a known reflectance standard. It helps in establishing a reliable scale for reflectance measurements across the spectrum.
- Multipoint Calibration: This is essential for spectrometers having multiple channels. By measuring several reference points across the range of interest, this method provides a more robust calibration curve.
Effective calibration can compensate for instrumental drift and variations in environmental conditions. Researchers must regularly calibrate their equipment to ensure high precision in their measurements, ultimately leading to more reliable data in their analysis.
Accurate calibration techniques are critical for achieving reliable and reproducible results in spectroscopic measurements.
Optical Techniques in Leaf Spectroscopy
The realm of leaf spectrometry is significantly enhanced by optical techniques that provide vital insights into plant physiology. Understanding the way light interacts with leaf material allows researchers to gather critical data regarding chlorophyll content, health status, and overall plant viability. This knowledge becomes essential when analyzing the biological responses to environmental variables and agricultural practices. By applying optical techniques, scientists can effectively monitor plant health and assess ecosystem dynamics.
Reflectance and Transmittance Measurements
Reflectance and transmittance measurements are two fundamental optical techniques used in leaf spectroscopy. Reflectance is the proportion of light that bounces off the leaf surface, while transmittance refers to the amount of light that passes through the leaf. Together, these measurements provide a comprehensive view of light absorption and utilization by plants.
- Importance of Reflectance:
Reflectance measurements can reveal important details about leaf pigment concentrations, especially chlorophyll. High reflectance in the near-infrared range indicates healthy chlorophyll absorption, allowing researchers to infer plant vitality. - Significance of Transmittance:
Transmittance measurements offer insights into leaf structure and thickness. A higher transmittance rate can indicate lower chlorophyll content, signaling stress or health issues. - Applications in Research:
- Environmental monitoring: Helps assess changes in plant communities due to climate change.
- Agriculture: Facilitates precision farming strategies by evaluating crop health.
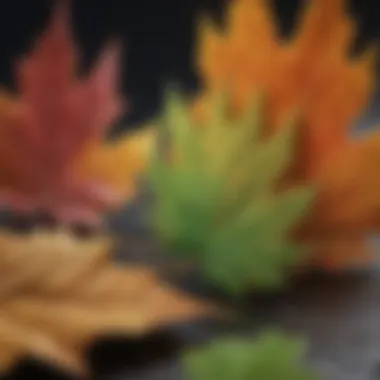
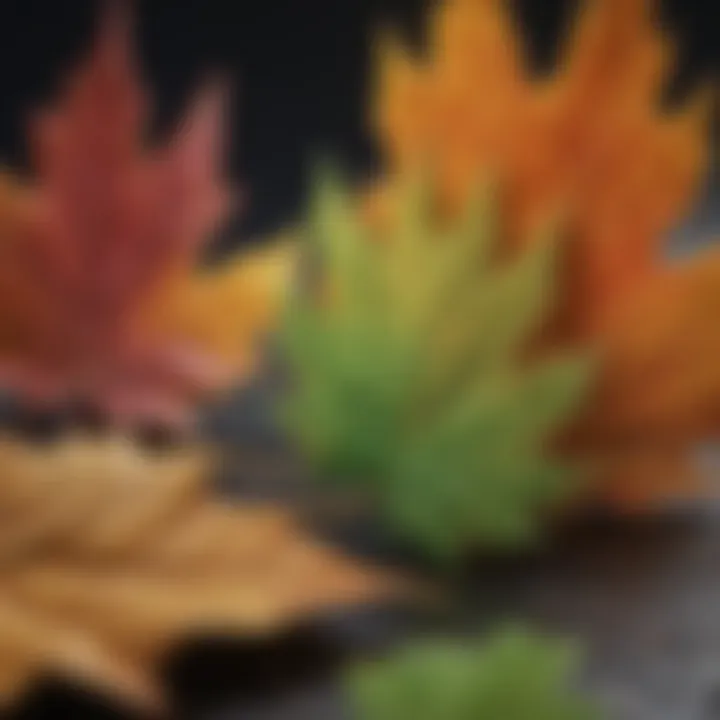
By evaluating both reflectance and transmittance, researchers gain a clearer picture of how plants interact with light, forming a bridge between optical analysis and practical application in both science and agriculture.
Fluorescence in Leaf Analysis
Fluorescence in leaf analysis is another crucial optical technique, where specific wavelengths of light excite chlorophyll molecules, causing them to emit light at longer wavelengths. This phenomenon can provide distinct insights into plant health and stress responses.
- Chlorophyll Fluorescence:
Chlorophyll fluorescence is a key measure of photosynthetic efficiency. Variations in fluorescence can indicate the level of stress a plant is experiencing, such as drought or nutrient deficiency. - Research Insights:
- Utilization of Technology:
Modern spectrometers equipped with fluorescence sensors enhance the ability to analyze leaf samples rapidly and accurately, improving the understanding of plant health.
- Photosynthetic performance: This technique is instrumental in determining how efficiently a plant converts solar energy into chemical energy.
- Stress detection: Early detection of stress through fluorescence can guide interventions in agriculture and conservation efforts.
In the context of leaf spectrometry, understanding fluorescence dynamics allows for monitoring plant health and predicting yield potential.
In summary, optical techniques such as reflectance, transmittance measurements, and fluorescence offer valuable tools in leaf spectrometry. They not only reveal critical information regarding plant health but also broaden the horizons of research in agriculture and environmental science.
Chlorophyll Absorption Spectra
Chlorophyll absorption spectra play a crucial role in understanding plant physiology and health. They provide insights into the biochemical composition of leaves, primarily focusing on chlorophyll types and their effective absorption wavelengths. This section aims to explicate the significance of chlorophyll absorption and its practical implications in various fields.
Understanding Chlorophyll Composition
Chlorophyll is the pigment responsible for the green color of plants. Its composition varies on a range, including chlorophyll-a and chlorophyll-b, each absorbing light differently. The absorption spectrum indicates the light wavelengths that chlorophyll can effectively capture for photosynthesis.
- Chlorophyll-a absorbs mainly in the blue (around 430 nm) and red (around 660 nm) regions.
- Chlorophyll-b absorbs light closer to the blue spectrum (around 450 nm) and slightly in the orange (around 640 nm).
This selective light absorption enhances the efficiency of photosynthesis by utilizing sunlight optimally. Understanding these absorption peaks is vital for researchers studying plant growth, energy conversion, and overall physiological health.
Implications for Plant Health Monitoring
Monitoring chlorophyll absorption spectra provides substantial benefits in assessing plant health. By analyzing the chlorophyll content, researchers can determine the photosynthetic capacity and vigor of plants. The following points highlight its implications:
- Nutritional Status: Chlorophyll levels can indicate nutrient deficiencies in plants. Low levels of chlorophyll may suggest a lack of essential nutrients such as nitrogen.
- Stress Detection: Changes in chlorophyll absorption can signal abiotic stress, including drought or salinity.
- Yield Prediction: Studies show a correlation between chlorophyll reading and crop yield, thus enabling better forecasting of agricultural outputs.
"Chlorophyll measurements serve as non-invasive indicators for evaluating crop health and vitality, thus enhancing decision-making in agriculture."
Applications in Agriculture
The application of leaf spectrometry in agriculture presents numerous advantages that significantly enhance the way we manage and optimize crop production. Through the analysis of leaf reflectance and absorption, farmers and agronomists obtain a clearer understanding of plant health and its needs. This data-driven approach allows for more precise decision-making, which is essential in todayβs increasingly competitive agricultural landscape. Key elements include better resource management, tailored interventions, and enhanced yields.
Precision Agriculture Techniques
Precision agriculture relies on accurate data to improve crop management. Leaf spectrometers play a vital role in this realm, enabling the collection of specific information about plant health in real time. By employing leaf spectrometry, farmers can achieve the following:
- Nutrient Management: Spectrometers can detect nutrient deficiencies early, allowing farmers to apply fertilizers more effectively. This targeted application reduces waste and environmental impact.
- Water Management: Understanding leaf water content through spectral analysis helps in scheduling irrigation. This precision minimizes water use while maximizing crop health.
- Variable Rate Technology: This technique allows for the application of inputs, like seeds or fertilizers, to be adjusted according to the exact needs of different areas of a field. Leaf spectrometry provides the necessary data for such adjustments.
These technologies not only enhance productivity but also promote sustainability in agricultural practices, aligning with modern environmental goals.
Disease Detection in Crops
The capability of leaf spectrometry to aid in disease detection is particularly noteworthy. Early detection of crop diseases can be the difference between maintaining a healthy field and incurring substantial losses. Leaf spectrometers identify unique spectral signatures that indicate stress or the presence of pathogens. Key benefits include:
- Rapid Diagnosis: By analyzing leaf samples, farmers can quickly diagnose diseases that often go unnoticed until significant damage has occurred.
- Specificity of Detection: Different diseases have distinct spectral responses. Leaf spectrometry can differentiate between types of diseases, allowing for targeted treatment strategies.
- Predictive Analysis: Historical data on spectral responses can help predict potential outbreaks. This forecasting enhances proactive disease management strategies.
The integration of leaf spectrometry into agricultural practices revolutionizes how we understand and respond to plant health challenges.
In summary, the applications of leaf spectrometry in agriculture are numerous and vital. They enhance precision techniques and disease detection, ultimately optimizing resource use and increasing overall productivity.
Role in Environmental Science
The role of leaf spectrometry in environmental science cannot be overstated. This technology provides vital information about ecosystem health, plant physiology, and the impact of external factors, such as climate change, on vegetation. Understanding these aspects is essential for researchers and policymakers alike, as it aids in the development of sustainable practices and informed decision-making.
Monitoring Ecosystem Health
Monitoring ecosystem health is a critical task in environmental science. Leaf spectrometers play a key role in assessing the vitality of plant communities. They can identify changes in plant health early, allowing for timely intervention. For instance, by analyzing the absorption and reflectance spectra of leaves, scientists can determine the concentration of chlorophyll and other pigments. These measurements indicate the photosynthetic efficiency and overall health of plants.
- Chlorophyll Levels: Monitoring chlorophyll content helps understand how well plants are photosynthesizing. A decrease in chlorophyll levels may suggest stress due to environmental factors, such as drought or nutrient deficiency.
- Species Diversity: Spectrometry allows researchers to distinguish between different plant species and monitor biodiversity within ecosystems. This information is vital for conservation efforts and ecosystem management.
- Soil and Water Quality: Changes in leaf reflectance can also correlate with soil moisture levels and nutrient availability. Thus, leaf spectrometry acts as a proxy for assessing environmental conditions.
"Leaf spectrometry serves as a window into the health of ecosystems, allowing us to detect changes before they become critical."
Assessing Impact of Climate Change
Assessing the impact of climate change is another significant application of leaf spectrometry. Plants respond to changes in temperature, precipitation, and atmospheric composition. Monitoring how these factors affect leaf characteristics can provide insights into broader ecological shifts.
- Phenological Changes: Spectrometry can help track changes in phenology, such as flowering times and leaf fall. Shifts in these patterns can signal how climate change is altering ecosystems.
- Carbon Sequestration: By understanding the photosynthetic capacity of plants, researchers can estimate their ability to capture carbon dioxide. This is crucial for evaluating the role of vegetation in mitigating climate change.
- Predicting Future Impacts: Continuous monitoring with leaf spectrometers can help predict how ecosystems might respond to future climate trends, aiding in the development of adaptive strategies.
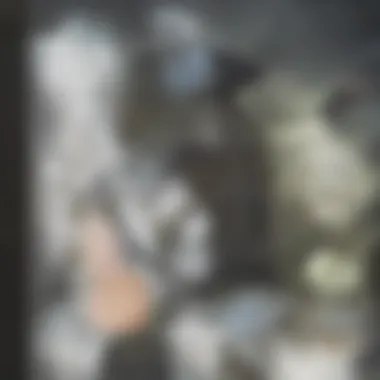
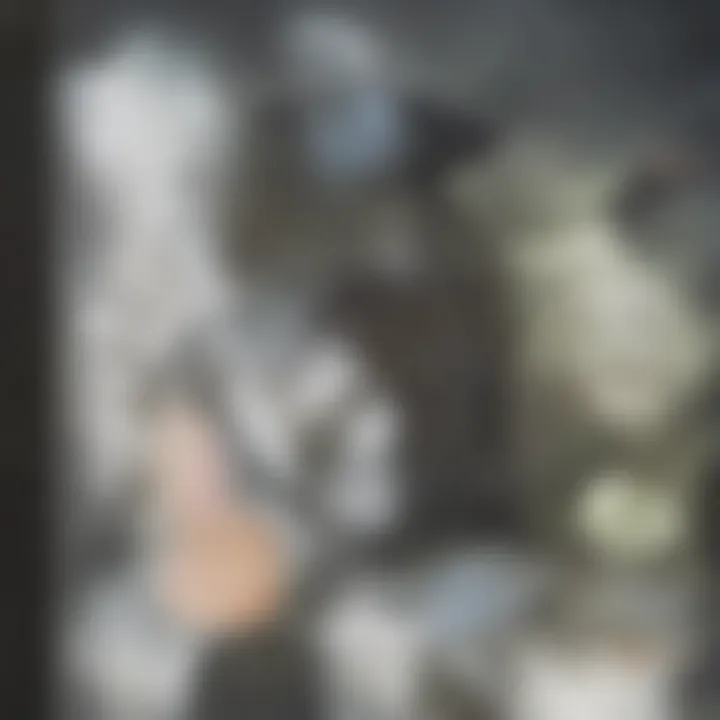
In summary, leaf spectrometers are indispensable tools in environmental science. They provide essential data that helps monitor ecosystem health and analyze the impacts of climate change. By leveraging this technology, researchers can gain deeper insights into plant responses and ecosystem dynamics.
Integration with Remote Sensing Technologies
The integration of leaf spectrometry with remote sensing technologies represents a significant advancement in our capacity to collect and analyze data regarding ecosystem health. This fusion allows for broader spatial coverage and the capability to monitor plant health over large areas. By leveraging satellite imagery and aerial surveillance, it becomes possible to gather spectral data that was previously inaccessible, enabling researchers to map vegetation conditions efficiently.
Combining Leaf Spectrometry with Satellite Data
Combining leaf spectrometry with satellite data offers a multi-faceted approach to understanding the complexities of plant ecosystems. Satellites like Landsat and Sentinel provide a wealth of information through various spectral bands that reflect different aspects of plant health, including chlorophyll content and moisture levels.
Using leaf spectrometry, data from these satellites can be calibrated to give more accurate field-level assessments. The integration provides higher resolution results. For example, spectral indices such as the Normalized Difference Vegetation Index (NDVI) can be enhanced by the detailed information gleaned from leaf spectrometers. This kind of combined analysis can reveal stress levels in plants more effectively than using satellite data alone.
Advancements in Data Analysis
Data analysis tools have progressed significantly in recent years, allowing for more complex interpretations of the data generated through remote sensing and leaf spectrometry. Advanced algorithms and machine learning techniques have been developed to process vast quantities of spectral data. These tools enable the identification of subtle changes in plant health over time and across different environmental conditions.
In particular, the use of deep learning models can improve the accuracy of predictions regarding plant stress and productivity. The coupling of in situ leaf spectrometer measurements with remotely sensed data produces richer datasets that can inform agricultural practices and ecological studies.
Additionally, cloud computing offers scalable solutions to handle the large datasets created by these technologies. This ensures that researchers can analyze data more quickly and efficiently, enabling timely decision-making.
The integration of remote sensing with leaf spectrometry not only enhances our understanding of plant dynamics but also paves the way for innovative approaches to sustainable management of natural resources.
Limitations and Challenges
In understanding the capabilities and applicability of leaf spectrometers, it is important to address their limitations and challenges. Despite their advanced technology and broad usability, leaf spectrometers face several constraints that can influence their performance and the accuracy of their results. Recognizing these challenges helps in better interpreting data derived from these instruments and applying it effectively in research and practical applications.
Physical Limitations of Spectrometers
Leaf spectrometers are sophisticated devices designed to analyze the spectral properties of plants. However, they carry intrinsic physical limitations that can affect their overall accuracy and usability. Some significant limitations include:
- Wavelength Range: Not all leaf spectrometers cover the full spectrum of wavelengths. Certain instruments may miss critical absorbance peaks that are vital for specific analyses, hence could lead to incomplete or skewed results.
- Resolution: The spectral resolution determines how finely a spectrometer can distinguish between different wavelengths. A low resolution may not resolve closely spaced spectral features, resulting in poor data clarity.
- Sensitivity: Spectrometers differ in their sensitivity to light intensity. Devices with lower sensitivity might struggle in low-light conditions, impacting the accuracy of the measurements.
- Data Processing Limitations: The algorithms used for interpreting spectral data can also limit what can be deduced from the data obtained. Simplistic models might overlook complex interactions that affect leaf spectral responses.
Addressing these limitations requires careful planning during the selection of spectrometers based on the specific research needs. A comprehensive understanding of the physical constraints ensures reliable methodology in research.
Environmental Factors Affecting Measurements
Environmental conditions significantly influence the performance of leaf spectrometers. Factors such as light quality, temperature, and humidity can alter the measurements, affecting data reliability. The following elements warrant consideration:
- Light Quality: Spectral measurements can be influenced by the type of light under which samples are analyzed. Variations in background light can introduce noise, affecting the spectral readings.
- Temperature Fluctuations: Changes in temperature can lead to shifts in the structural properties of leaf tissues, which might affect their optical characteristics. It's essential to control or minimize temperature variability during data collection.
- Humidity: High humidity levels can cause condensation on optical components, leading to erroneous measurements. Controlling moisture levels in the measurement environment is crucial to obtaining accurate data.
- Surface Conditions of Leaves: The presence of dirt, water, or other contaminants on leaf surfaces can distort the spectral data. Ensuring clean leaf surfaces can mitigate this issue, so meticulous sample preparation is crucial.
Proper attention to these environmental factors can enhance the reliability of measurements obtained from leaf spectrometers. Researchers must be aware of these elements to design effective experiments and validate their findings in the context of leaf physiology and ecosystem health.
Future Trends in Leaf Spectrometry
Examining the future trends in leaf spectrometry is essential for understanding how this field may evolve and impact related disciplines. As technology progresses, methods of measuring leaf properties and processes will likely become more efficient and accurate. Enhanced leaf spectrometers will play a significant role in agricultural practices, environmental monitoring, and scientific research.
Emerging Technologies
New advancements in technology continually shape the capabilities of leaf spectrometers. Some of the most notable emerging technologies include:
- Miniaturization: Modern spectrometers are becoming smaller and more portable. Handheld devices allow for field measurements, increasing accessibility for researchers and farmers.
- Hyperspectral Imaging: This technique captures a wide spectrum of light across many wavelengths. It provides detailed information about plant health and stress responses.
- Artificial Intelligence Integration: The use of AI in data analysis offers the ability to process large datasets more effectively. Machine learning algorithms may identify patterns in leaf spectra that humans could miss.
- Unmanned Aerial Vehicles (UAVs): Drones equipped with spectrometers are increasingly used to survey large agricultural areas quickly. This allows for real-time data collection from inaccessible locations.
These technologies promise to enhance the precision and application of spectrometry, making it a vital tool in plant research and management.
Potential Research Areas
Several significant research directions are anticipated in leaf spectrometry, warranting further exploration and investment. Potential areas of study include:
- Plant Stress Responses: Understanding how leaves respond to various stressors like drought or pests through spectral analysis can help improve crop resilience.
- Biodiversity Monitoring: Measuring leaf spectral data can aid in assessing plant biodiversity in ecosystems, informing conservation efforts.
- Nutrient Management: Research into leaf nutrient content through spectrometry can facilitate tailored fertilization strategies, improving yield and reducing environmental impact.
- Climate Change Impact Assessment: Evaluating how climate change affects leaf characteristics can contribute valuable insights into ecosystem shifts and plant adaptability.
These areas present exciting opportunities for researchers and practitioners. By addressing these topics, experts can further understand plant dynamics and enhance agricultural practices to adapt to a changing world.
The End
In summary, the topic of leaf spectrometry holds critical importance in the realms of scientific research and practical application. By analyzing leaf spectra, researchers can gain insights into plant physiology, ecosystem dynamics, and environmental health. This article emphasizes several specific elements that underline the value of leaf spectrometers in various fields.
Summarizing Key Findings
Leaf spectrometry enables the detailed study of chlorophyll absorption, providing crucial data on plant health and productivity. Key findings from this analysis include:
- Enhanced Understanding of Plant Physiology: Through the understanding of chlorophyll and its spectral characteristics, researchers can assess the condition of plants effectively.
- Applications in Agriculture: The integration of leaf spectrometry into agriculture has led to precise monitoring of crop health and yield prediction, which can contribute to better management practices.
- Environmental Monitoring: Leaf spectrometry serves as a robust tool for monitoring ecosystem health and the effects of climate change on plant communities.
- Technological Advancements: Recent improvements in spectrometer design and data analysis techniques have expanded the capabilities and accuracy of measurements, leading to refined research outcomes.
Leaf spectrometry is not just a technique; it allows for comprehensive insights that bridge the gap between plant physiology and environmental science.
Implications for Future Research
Looking ahead, the future of leaf spectrometry appears promising, with various implications for ongoing and upcoming research initiatives:
- Emerging Technologies: The advent of more portable and efficient spectrometers will make it easier to conduct field studies, fostering a deeper understanding of plant responses in natural settings.
- Interdisciplinary Research Areas: There is an increasing need for studies that combine leaf spectrometry with other scientific fields, such as genetics and ecology, to uncover complex interactions in ecosystems.
- Long-term Ecosystem Monitoring: As climate change continues to impact global ecosystems, long-term leaf spectrometry studies will be vital for assessing changes in vegetation and its overarching effects on biodiversity.
Advancing our understanding through leaf spectrometry will pave the way for innovative agricultural practices and enhance our strategies for environmental conservation.